Yushi Zhao, Wenjun He, Zhiying Liu, Yuegang Fu. Development of convex blazed grating in coded aperture spectral imager[J]. Infrared and Laser Engineering, 2022, 51(3): 20220007

Search by keywords or author
- Infrared and Laser Engineering
- Vol. 51, Issue 3, 20220007 (2022)
Abstract
(1) |
View in Article
(2) |
View in Article
(3) |
View in Article
(4) |
View in Article
(5) |
View in Article
(6) |
View in Article
(7) |
View in Article
(8) |
View in Article
(9) |
View in Article
(10) |
View in Article
(11) |
View in Article
(12) |
View in Article
(13) |
View in Article
(14) |
View in Article
(15) |
View in Article
(16) |
View in Article
(17) |
View in Article
(18) |
View in Article
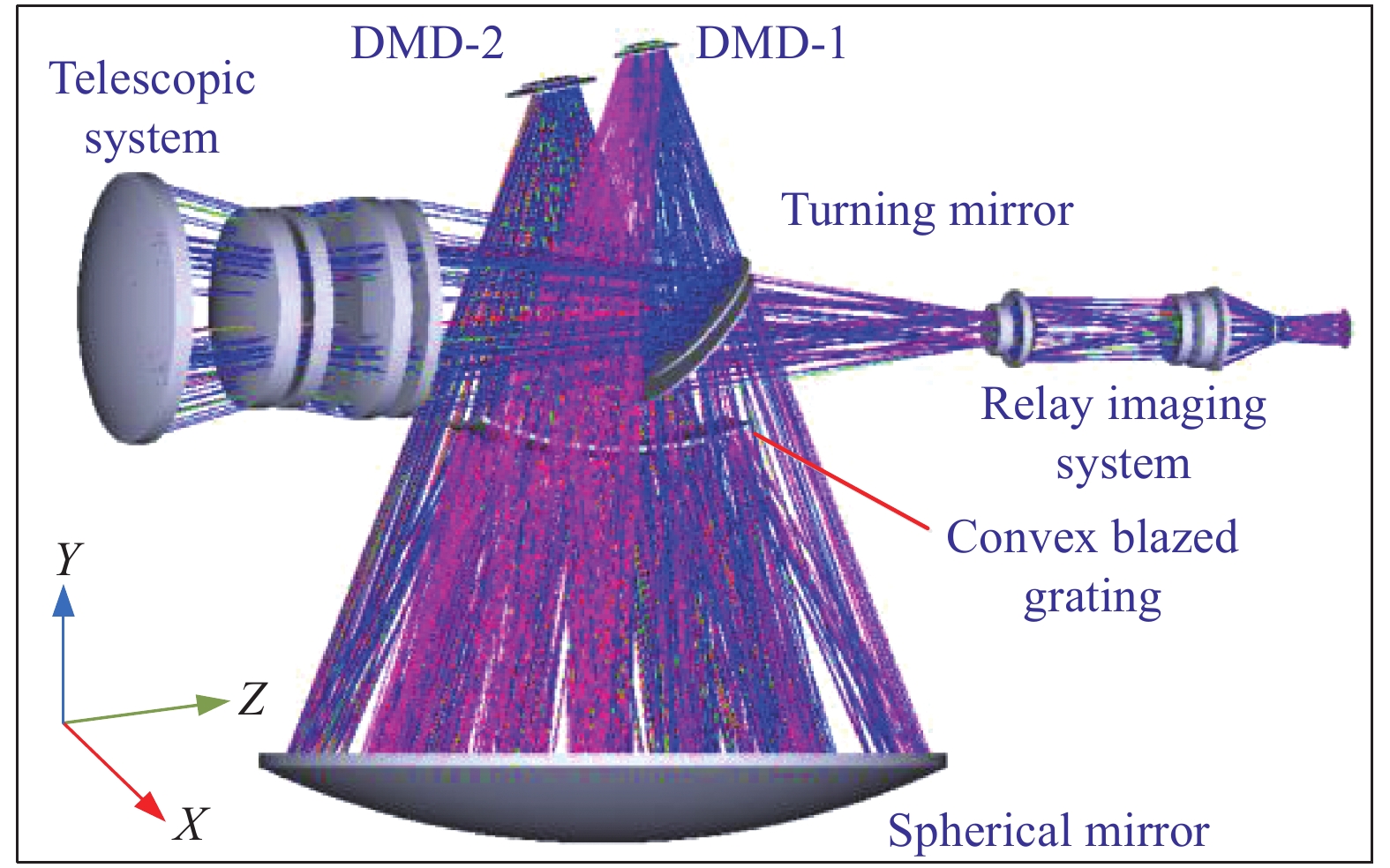
Set citation alerts for the article
Please enter your email address