Esrom Kifle, Pavel Loiko, Javier Rodríguez Vázquez de Aldana, Carolina Romero, Airán Ródenas, Sun Yung Choi, Ji Eun Bae, Fabian Rotermund, Viktor Zakharov, Andrey Veniaminov, Magdalena Aguiló, Francesc Díaz, Uwe Griebner, Valentin Petrov, Xavier Mateos, "Passively Q-switched femtosecond-laser-written thulium waveguide laser based on evanescent field interaction with carbon nanotubes," Photonics Res. 6, 971 (2018)

Search by keywords or author
- Photonics Research
- Vol. 6, Issue 10, 971 (2018)
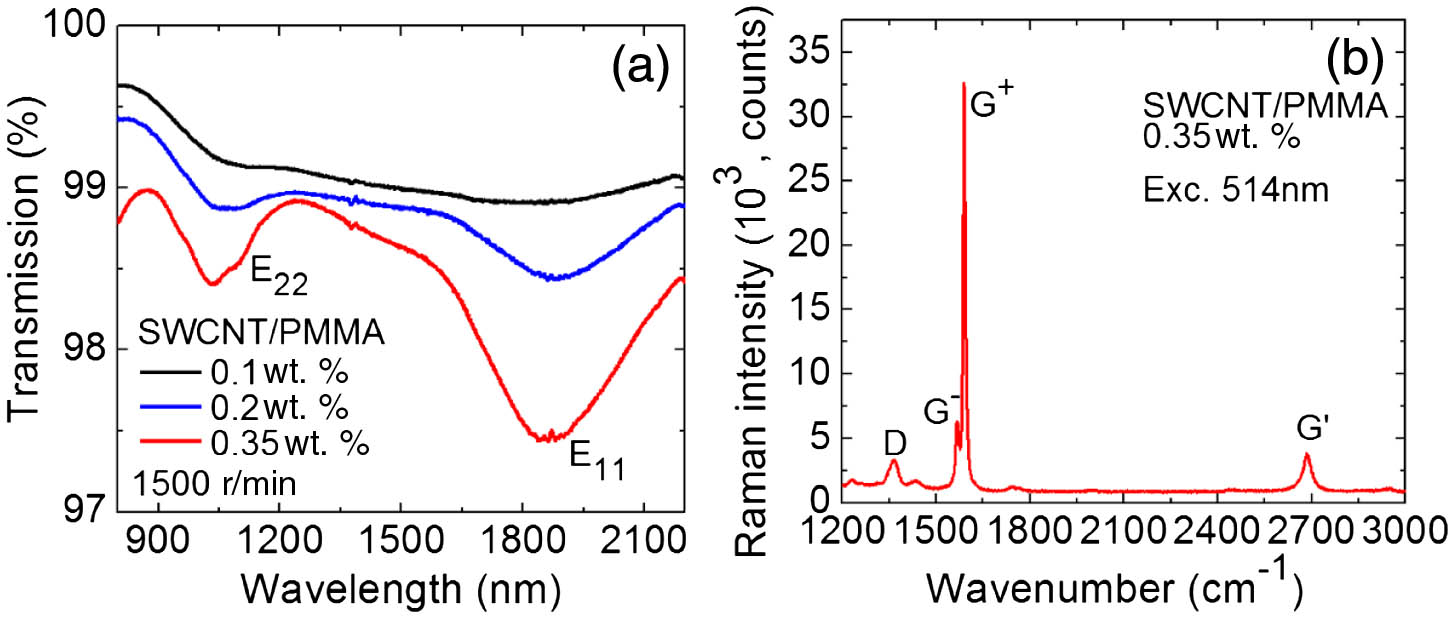
Fig. 1. (a) Small-signal internal transmission spectra of the SWCNT/PMMA films coated on quartz substrates with different concentrations of SWCNTs (0.1–0.35 wt. %); the spin-coating speed is 1500 r/min. (b) Raman spectrum of the 0.35 wt. % SWCNT/PMMA film coated on the surface of Tm:KLuW, λ exc = 514 nm .
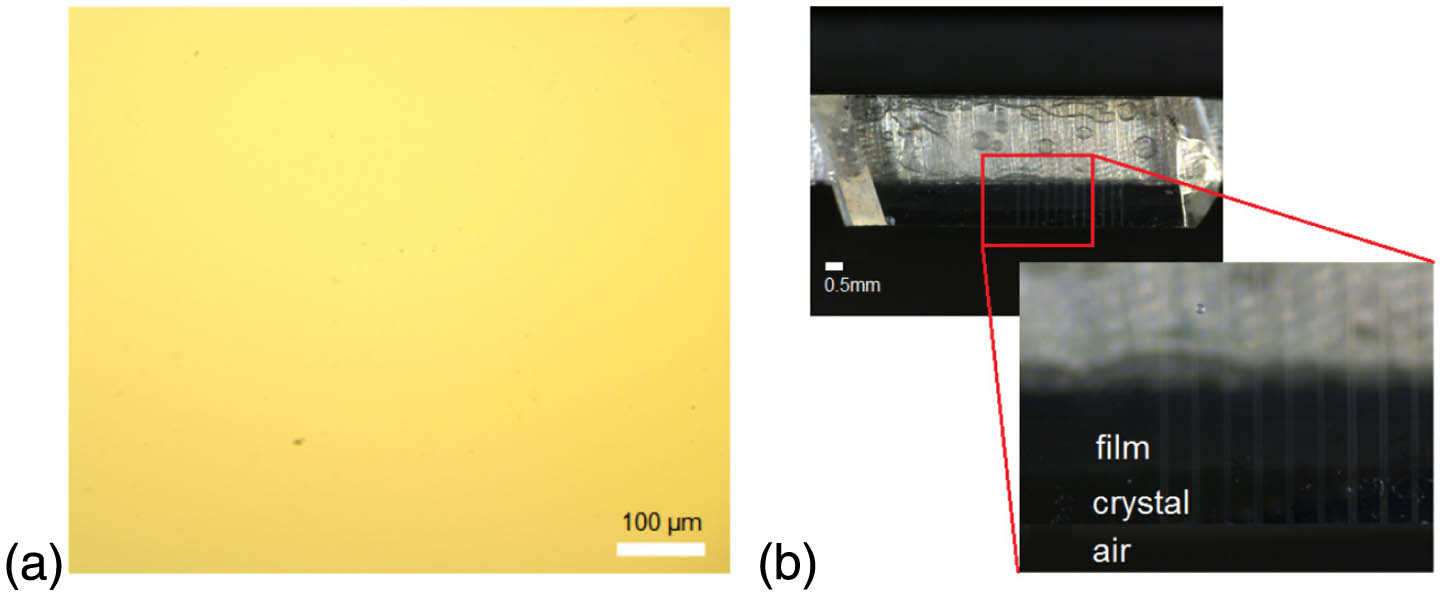
Fig. 2. (a) Optical microscope image of the surface of SWCNT/PMMA film (200 × magnification); (b) photograph of the Tm:KLuW crystal containing surface channel WGs with the deposited SWCNT/PMMA film.
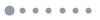
Fig. 3. Confocal laser microscopy of a polished end-face of the fs-DLW Tm:KLuW surface channel WGs with cladding diameters of (a) 60 μm and (b) 50 μm, transmission mode, polarized light (P | | N p ), λ = 405 nm .
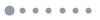
Fig. 4. Confocal laser microscope images (top view) of the central part of the half-ring cladding fs-DLW surface WGs in Tm:KLuW: (a), (b) transmission mode, polarized light (P | | N g ), λ = 405 nm ; (c), (d) transmission mode, crossed polarizers (P | | N g , A | | N m ), λ = 488 nm . WGs: (a), (c) 60 μm cladding and (b), (d) 50 μm cladding.
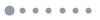
Fig. 5. μ -Raman mapping of a lateral face of the fs-DLW Tm:KLuW surface channel WGs monitoring the ∼ 907 cm − 1 Raman band: (a), (c) peak intensity (a.u.) and (b), (d) peak frequency (cm − 1 ). WGs: (a), (b) 60 μm cladding and (c), (d) 50 μm cladding. The measurement geometry is g ( m m ) g . The N m -axis is horizontal.
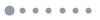
Fig. 6. (a) Scheme of the PQS fs-DLW Tm:KLuW surface WG laser: ND, gradient neutral density filter, PM, pump mirror, WG, waveguide, OC, output coupler, F, cutoff filter. (b) Photograph of the Ti:sapphire pumped WG showing blue upconversion luminescence.
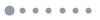
Fig. 7. Input–output dependences for CW fs-DLW Tm:KLuW surface channel WG lasers with (a) 60 μm and (b) 50 μm cladding, η –slope efficiency. The laser polarization is E | | N m .
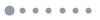
Fig. 8. Typical laser emission spectra of CW fs-DLW Tm:KLuW surface channel WG lasers with (a) 60 μm and (b) 50 μm cladding (measured at maximum P abs , Fig. 4 ). The laser polarization is E | | N m .
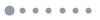
Fig. 9. Modified Caird analysis for 60 and 50 μm cladding CW fs-DLW Tm:KLuW surface channel WG lasers (symbols: experimental data; lines: their linear fits).
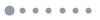
Fig. 10. Spatial near-field beam profiles of the output laser mode from the CW fs-DLW Tm:KLuW surface channel WG lasers with (a), (c) 60 μm and (b), (d) 50 μm cladding, T OC = 30 % , P abs = 0.4 W . The profiles were calibrated with respect to the WG output end-face. (a), (b) 2D profiles, (c), (d) intensity plots along the directions of the N m axis (horizontal) and the N p axis (vertical). Symbols: experimental data; curves: their Gaussian fits.
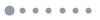
Fig. 11. Simulated fundamental mode for (a) 60 μm and (b) 50 μm WG. The DLW tracks and the crystal surface are indicated by red color. The assumed refractive index change at DLW tracks is − 0.01 + 0.0017 i .
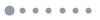
Fig. 12. fs-DLW Tm:KLuW surface channel WG lasers PQS by evanescent-field coupling with SWCNT-SA: (a) input–output dependences, η is slope efficiency; (b) typical laser emission spectra measured at maximum P abs . The laser polarization is E | | N m .
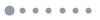
Fig. 13. Pulse characteristics of fs-DLW Tm:KLuW surface channel WG lasers PQS by evanescent-field coupling with SWCNT-SA: (a) pulse duration, (b) PRF, (c) pulse energy, and (d) peak power. T OC = 30 % .
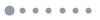
Fig. 14. Oscilloscope traces of (a), (b) the typical pulse trains and (c) the corresponding single Q -switched pulses for the fs-DLW Tm:KLuW surface channel WG lasers PQS by evanescent-field coupling with SWCNT-SA. WG lasers with (a), (c) 60 μm and (b), (c) 50 μm cladding, T OC = 30 % , P abs = 0.5 W .
|
Table 1. Pulse Characteristics of the fs-DLW Tm:KLuW Surface Channel Waveguide Lasers PQS by Evanescent-Field Coupling with SWCNTs
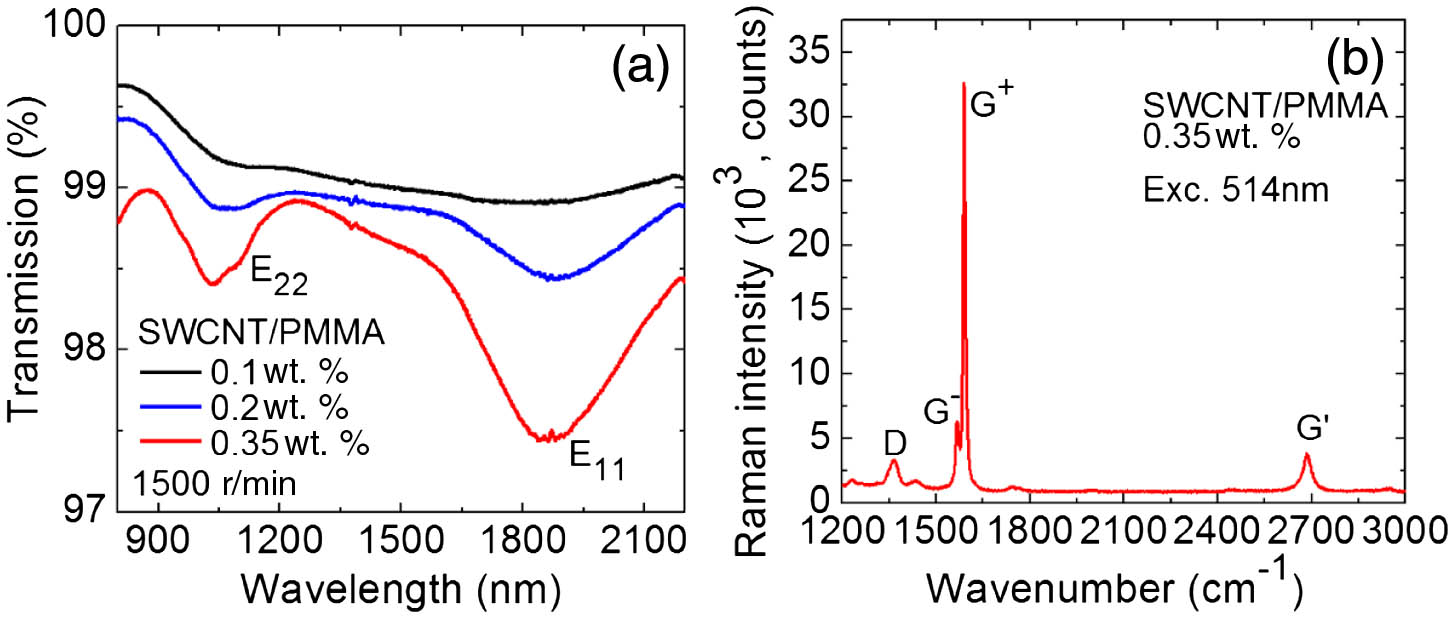
Set citation alerts for the article
Please enter your email address