Xiyu Lu, Yanjiao Guan, Pengchang Yang, Shan Niu, Yu Ma, Lijun Wang, Ning Zhuo, Jinchuan Zhang, Shenqiang Zhai, Fengmin Cheng, Shuman Liu, Fengqi Liu, Junqi Liu, "Dual-mode distributed feedback quantum cascade laser based on stacked 3D monolithic integration for on-chip multi-channel gas sensing," Photonics Res. 11, 2113 (2023)

Search by keywords or author
- Photonics Research
- Vol. 11, Issue 12, 2113 (2023)
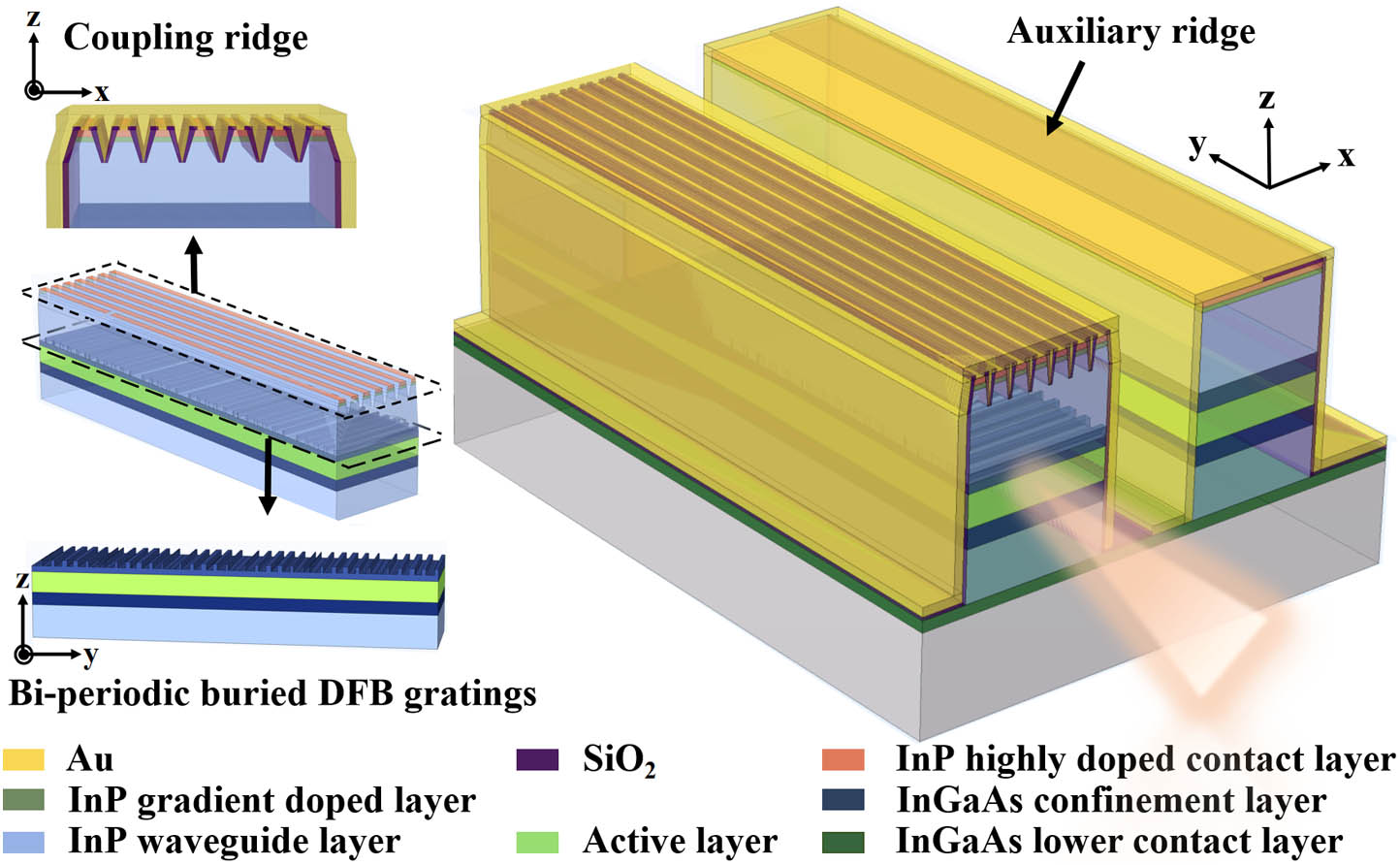
Fig. 1. Three-dimensional device structure diagram of coupled ridge dual-wavelength DFB QCL.

Fig. 2. (a) Electroluminescence spectrum of the materials. Δ E 1 and Δ E 2 represent the gain spectra from the upper energy level to the two lower energy levels, respectively. (b) Coupling coefficient varies with the grating duty cycle and grating depth for the two wavelengths. The upper insets show the calculated modal profiles of λ 1 emission with σ = 0.5 (left) and λ 2 emission with σ = 0.6 (right), respectively. (c) Schematic diagram of bi-period grating formed by different photolithographic stripes. (d) The propagation waves correspond to the gratings of the same color in Fig. 1 (c).
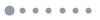
Fig. 3. Dependences of supermode loss and loss difference on (a) ridge period and (b) array etching depth at λ 1 = 7.5 μm . Fundamental supermode near-field profiles of the seven-element coupled ridge waveguide structure at (c) λ 1 = 7.05 μm and (d) λ 1 = 7.5 μm calculated using COMSOL finite element method software.
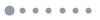
Fig. 4. (a) Cross-sectional photographs of the actual coupling ridge portions for parameter sets 1 (left) and 2 (right). (b) Cross-sectional photograph of actual device mounted epi-side down onto the graphite heat-sink.
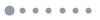
Fig. 5. (a) L - I - V curves at T sink from 10°C to 40°C for a 2-mm-long QCL with ridge width W S 1 = 7.5 μm . The inset shows J t h as a function of T in pulsed mode. The dashed line is the fitting result obtained using the exponential function J th = J 0 exp ( T / T 0 ) . (b) L - I - V curves at T sink from 20°C to 40°C for a 2-mm-long QCL with ridge width W S 2 = 8.9 μm .
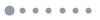
Fig. 6. Far-field profile along the ridge width direction at room temperature for a device with ridge period P 1 = 9.5 μm , ridge width W S 1 = 7.5 μm , and depth D 1 = 2.3 μm . (a) Before λ 2 lasing. (b) Lasing at dual wavelengths. Data fittings performed using the Gaussian function are represented by solid lines.
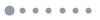
Fig. 7. (a) Optical spectra measured by varying the current at room temperature (20°C). (b) Emission spectra of the device during operation when T was varied from − 4 ° C to 60°C in increments of 4°C (measured at 1.05 × I th ). The insets show the linearly fitted tuning characteristics of lasing frequency versus injection temperature. (c) The upper part, spectrum of the tuning range combined with temperature and current tuning. The insets are single-mode spectra of the λ 1 and λ 2 emissions at 20°C with SMSR of over 20 dB. The lower part, the absorption lines of gas molecules within the tuning range.
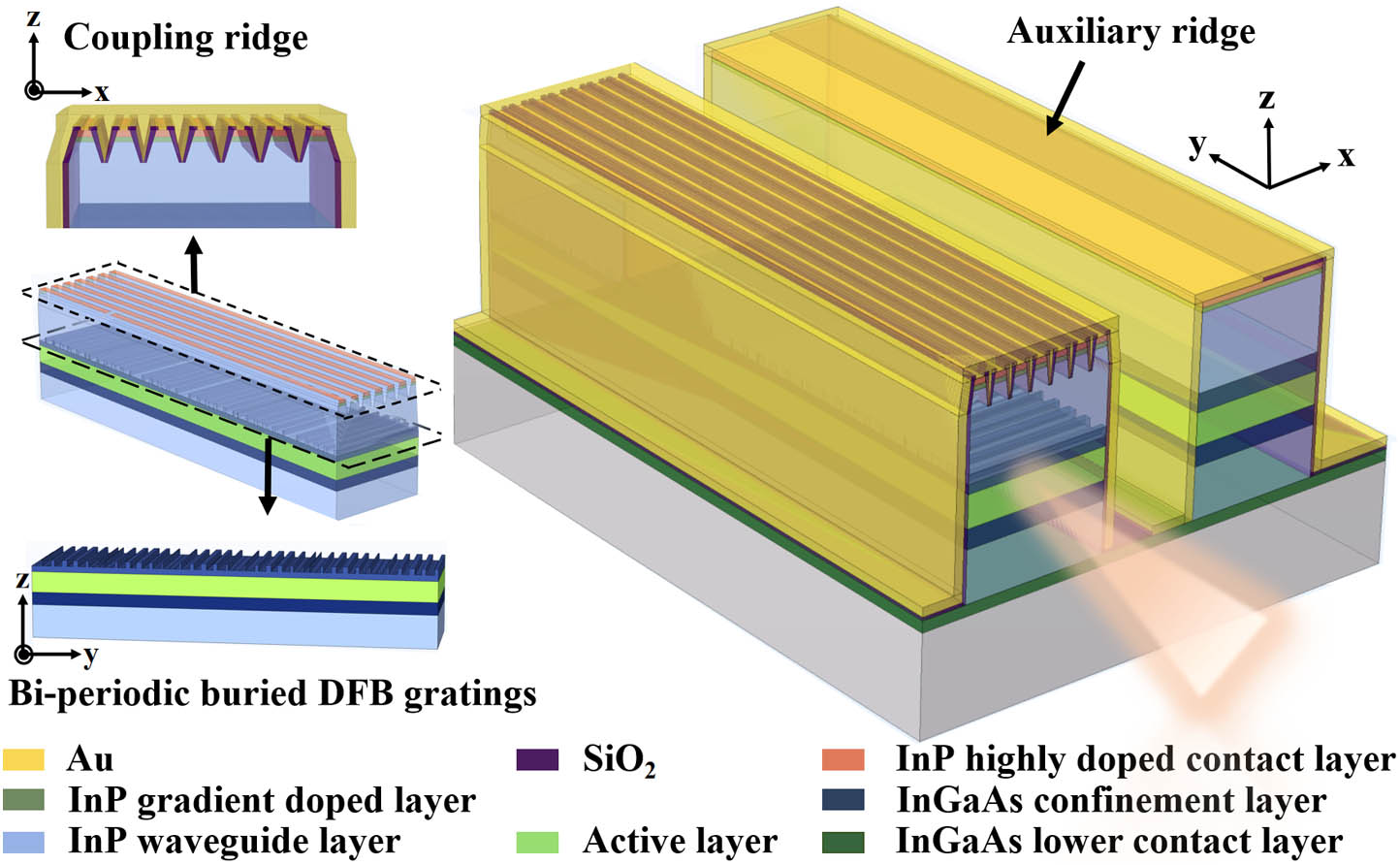
Set citation alerts for the article
Please enter your email address