Cuiping Ma, Peng Yu, Zhimin Jing, Yisong Zhu, Peihang Li, Wenhao Wang, Hongxing Xu, Yanning Zhang, Liang Pan, Tae-Youl Choi, Arup Neogi, Alexander O. Govorov, Zhiming Wang, "Circular polarization-selective optical, photothermal, and optofluidic effects in chiral metasurfaces," Photonics Res. 12, 331 (2024)

Search by keywords or author
- Photonics Research
- Vol. 12, Issue 2, 331 (2024)
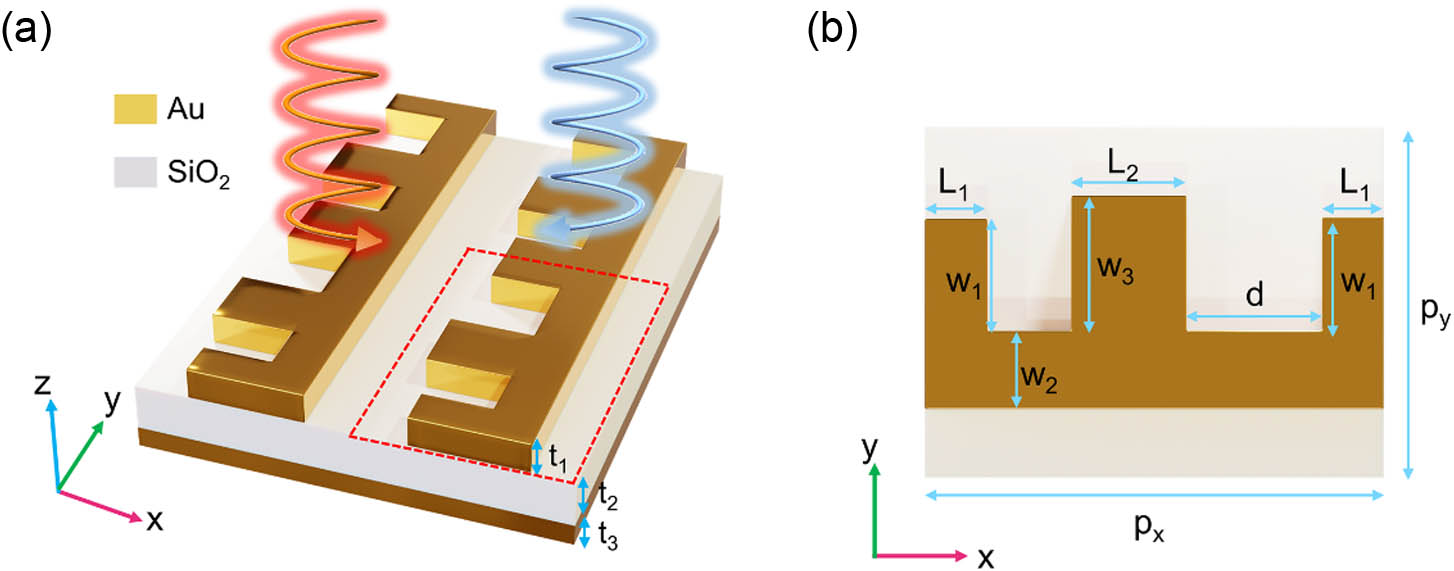
Fig. 1. Structure design of the metasurface. (a) Sketch of the chiral metasurface consisting of Au nanoantenna arrays and a bottom Au film separated by a SiO 2 layer. The substrate is silicon and the top medium is water. The thicknesses of the top Au resonators and bottom Au layer are t 1 = 55 nm and t 3 = 100 nm , respectively; the thickness of the SiO 2 spacer is t 2 = 150 nm . The red dashed box indicates the size of the unit cell. (b) Top view of the unit cell with a period of p x = 600 nm and p y = 470 nm . The optimized geometric parameters are set as L 1 = 75 nm , L 2 = 100 nm , w 1 = 180 nm , w 2 = 100 nm , w 3 = 200 nm , and d = 210 nm .
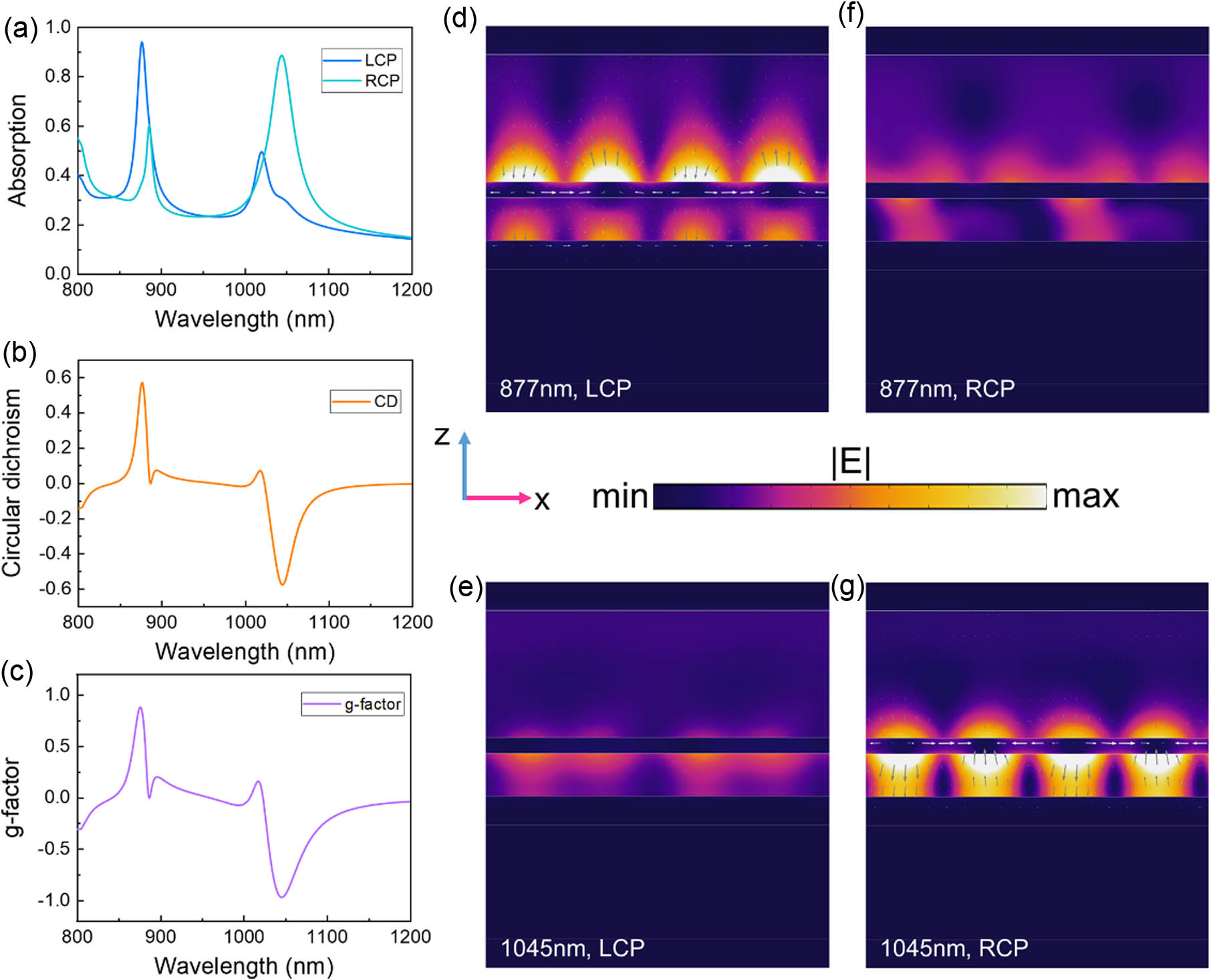
Fig. 2. Electromagnetic response of the chiral metasurface. (a) Simulated optical response under LCP (blue) and RCP (cyan) illumination. (b) CD and (c) g factor spectrum of the chiral metasurface. (d)–(g) Spatial electric intensity profiles are taken from the x z section at y = − 350 nm . The images are recorded at (d), (f) 877 nm and (e), (g) 1045 nm under (d), (e) LCP and (f), (g) RCP incidence.
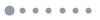
Fig. 3. Photothermal effect of the chiral metasurface. Average temperature increases of the whole system as a function of time under LCP (orange) and RCP (cyan) illumination at the resonant wavelength of (a) 877 nm and (b) 1045 nm. (c) Photothermal circular dichroism as a function of time at 877 nm (magenta) and 1045 nm (blue). (d)–(f) Photo-induced temperature increase in the x z section at y = 0 nm when t = 2000 ns . The data are recorded at (d) 877 nm and (e) 1045 nm under LCP (left) and RCP (right) illumination. (f) Spatial distribution of photothermal circular dichroism corresponding to (d) and (e).
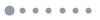
Fig. 4. Thermally-induced fluid motion of the water. (a) Velocity spectra with LCP (pink) and RCP (gray) illumination. (b) Calculated velocity CD (blue) and g v factor (purple) spectra. (c)–(h) Velocity patterns (double arrows) in the x z section at y = 0 nm . Other geometry is removed, and a false background highlights the fluid motion. Simulated fluid convection at (c), (d) 877 nm and (f), (g) 1045 nm under (c), (f) LCP and (d), (g) RCP illumination. (e), (h) Velocity CD profiles at 877 nm and 1045 nm, respectively. The data were recorded at t = 2000 ns .
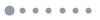
Fig. 5. Chiral fluid convection in y z section. (a)–(d) 3D temperature distribution maps in the resonator. The legend here only represents the temperature gradient instead of the actual temperature. (e)–(h) Velocity patterns (streamlines) overlaid the temperature gradient of the resonator in the y z section at x = 120 nm , as indicated by the red dashed line in the inset. (a), (b) and (e), (f) are recorded at the wavelength of 877 nm under (a), (e) LCP and (b), (f) RCP incidence. (c), (d) and (g), (h) are recorded at the wavelength of 1045 nm under (c), (g) LCP and (d), (h) RCP illumination.
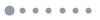
Fig. 6. g factor spectra. Optical absorption g factor (pink) and velocity g factor (gray) of the CA enantiomer. The inset shows the evolution of the photothermal g factor as a function of time in the CA (blue) and CB (purple) enantiomers.
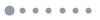
Fig. 7. (a) Simulated optical response of CB enantiomer under LCP (blue) and RCP (cyan) illumination. (b) CD spectrum of CB system. (c) g factor spectrum of CB system.
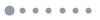
Fig. 8. Three-dimensional cross-section diagram of the electromagnetic response at the two wavelengths (a), (b) 877 nm and (c)–(e) 1045 nm under (a), (b) LCP and (c)–(e) RCP incidence. Simulated electric intensity profiles are taken from the x y section at (a), (d) z = − 100 nm and (b), (e) z = 100 nm . (c) Spatial electric intensity profiles taken from the y z section at x = 0 nm .
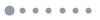
Fig. 9. Photo-induced temperature increase at the two wavelengths (a), (b) 877 nm and (c), (d) 1045 nm in the vertical cross-sections at y = 0 nm , as the red dashed line in the inset shows. (a), (c) and (b), (d) are recorded under LCP and RCP incidence, respectively. The incident light flux is I 0 = 10 5 W / cm 2 . The data are given at the time of t = 2000 ns .
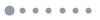
Fig. 10. Velocity patterns (streamlines) overlaying the temperature gradient of the resonator in x z -section at y = 100 nm , as indicated by the red dashed line in the inset. (a), (b) and (c), (d) are recorded at the wavelengths of 877 nm and 1045 nm under (a), (c) LCP and (b), (d) RCP incidence.
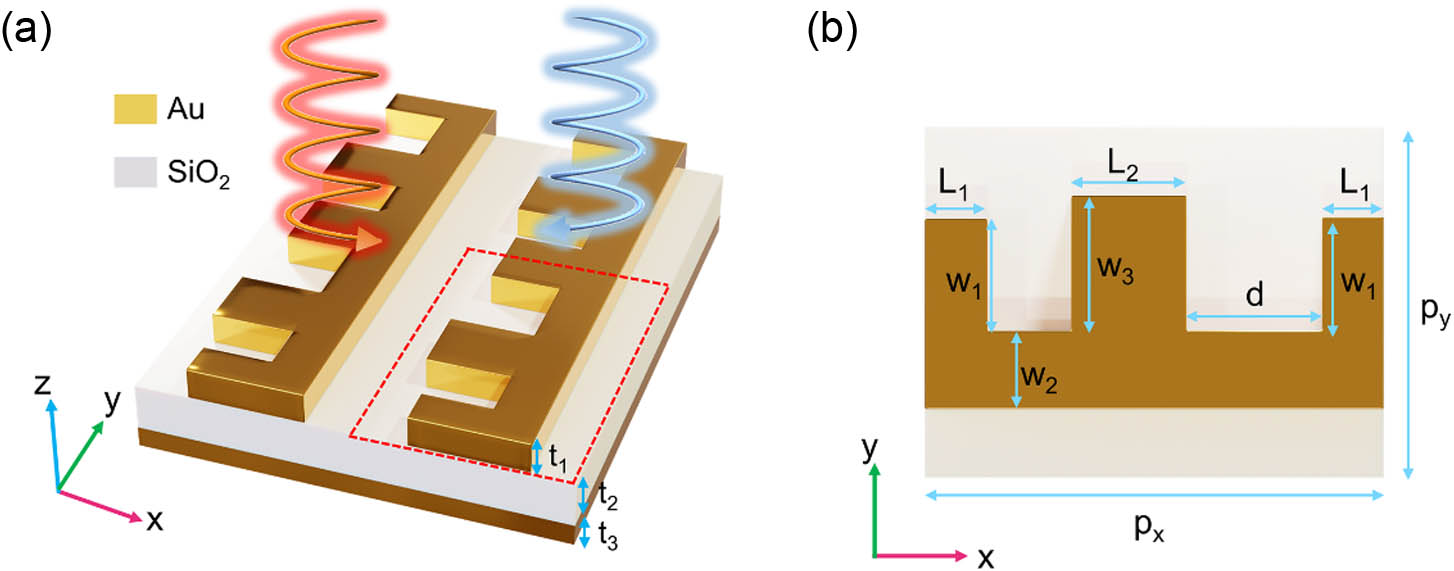
Set citation alerts for the article
Please enter your email address