Qianyi Mu, Fei Fan, Sai Chen, Shitong Xu, Chuanzhong Xiong, Xin Zhang, Xianghui Wang, Shengjiang Chang, "Tunable magneto-optical polarization device for terahertz waves based on InSb and its plasmonic structure," Photonics Res. 7, 325 (2019)

Search by keywords or author
- Photonics Research
- Vol. 7, Issue 3, 325 (2019)
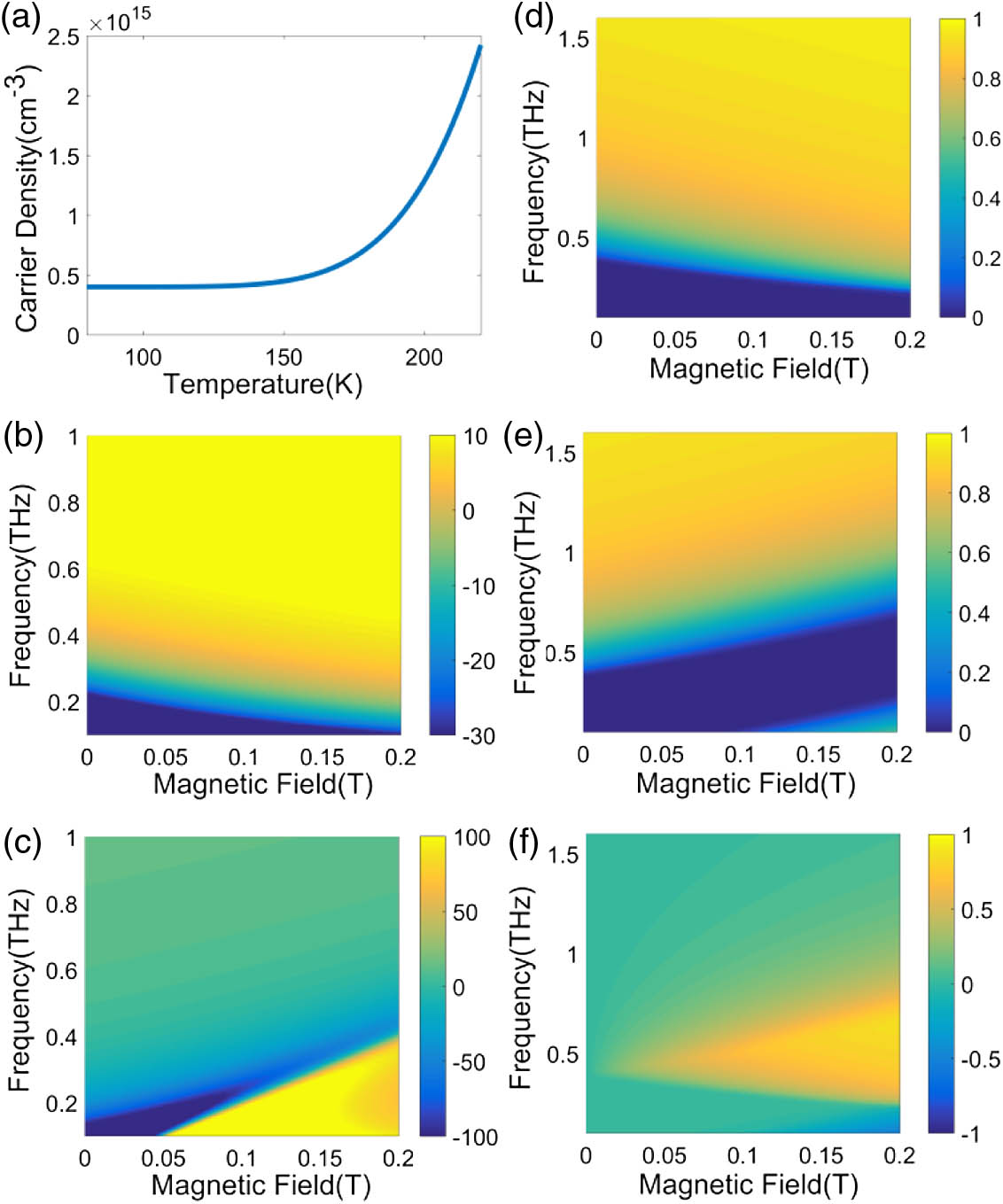
Fig. 1. (a) Simulative carrier density of InSb at different temperatures; maps of the real part of (b) ε L and (c) ε R of longitudinally magnetized InSb in the THz regime under different magnetic fields from 0 T to 0.2 T; maps of theoretical transmittance (d) I L and (e) I R of longitudinally magnetized InSb in the THz regime under different magnetic fields from 0 T to 0.2 T; (f) map of the theoretical transmittance difference between the LCP and the RCP (I L − I R ).
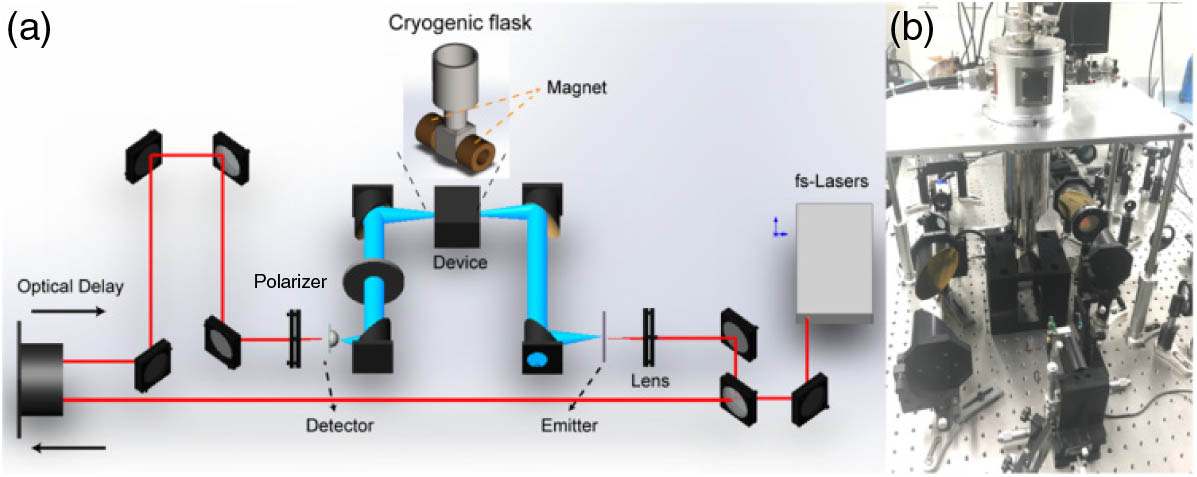
Fig. 2. (a) Schematic diagram of experimental THz-MOS system; (b) photo of the experimental equipment.
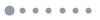
Fig. 3. Experimental and simulated results of InSb with different temperatures: (a) measured THz time domain pulses; (b) experimental intensity transmission expressed in dB; (c) simulated carrier density N and cutting frequency f c ; (d) simulated transmission.
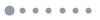
Fig. 4. Experimental results of InSb under magnetic field: (a) schematic diagram of the experimental configuration; (b) experimental time domain pulses in two orthogonal directions under magnetic fields of 150 mT and 0 mT; (c) experimental transmission of LCP and RCP components; (d) experimental Faraday rotation angles under different magnetic fields.
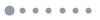
Fig. 5. Polarization state vectors of the transmitted THz wave through InSb when the input wave is an LP light: polarization state at (a) 0.7 THz and (b) 1.1 THz under different magnetic fields; polarization state under (c) 0.13 T and (d) 0.17 T at different frequencies.
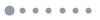
Fig. 6. (a) 3D schematic diagram of the InSb plasmonics in the experimental configuration; microscope image of grating 1 and grating 2; (b) side view of InSb plasmonics.
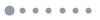
Fig. 7. Experimental results of the InSb plasmonics: (a) measured y -LP THz pulses under different magnetic fields; (b) THz pulses under forward and backward magnetic fields of 150 mT; (c) amplitude transmission spectra under different magnetic fields; (d) spectra of the extinction ratio.
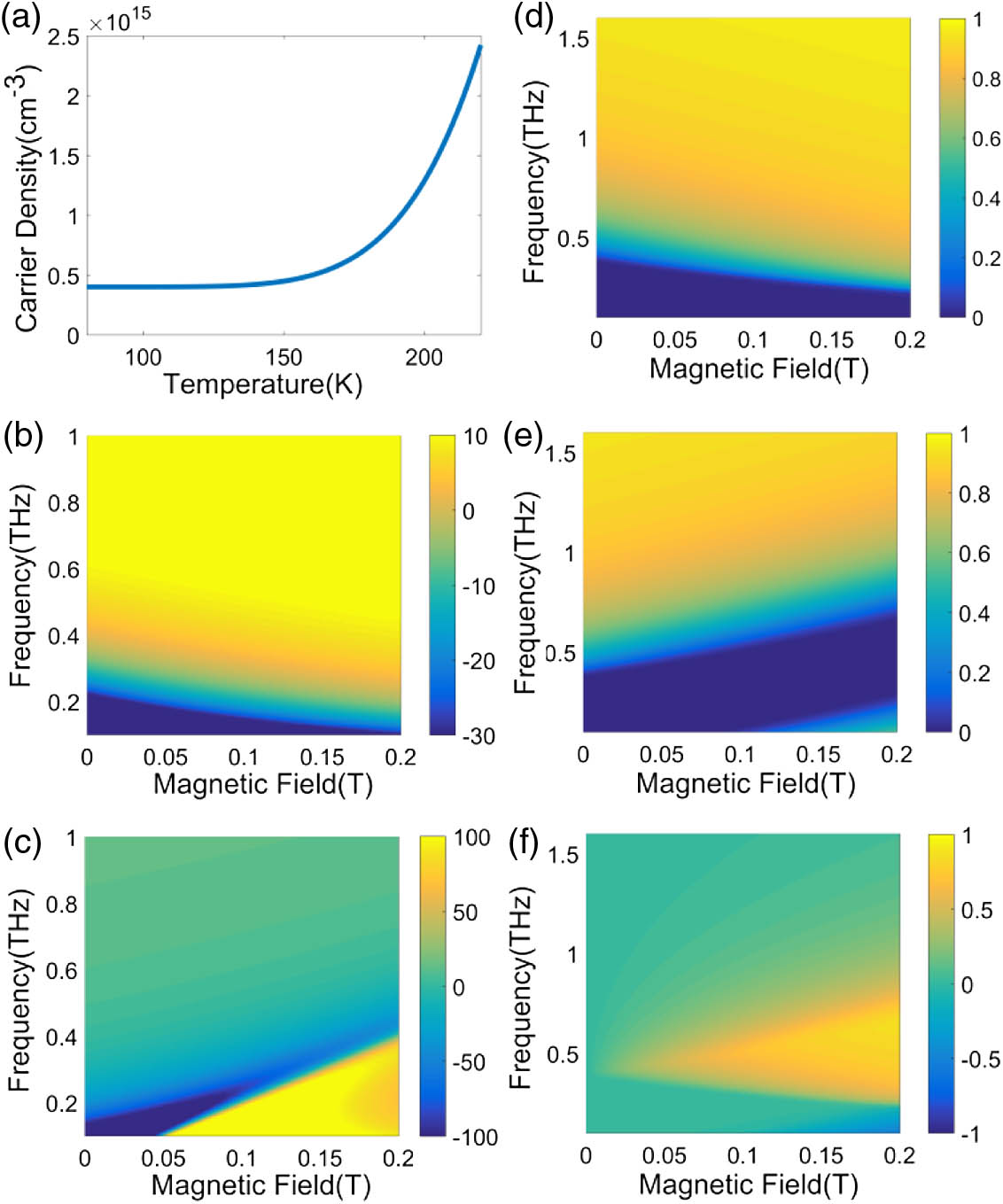
Set citation alerts for the article
Please enter your email address