Abstract
High performance electro-optic modulator, as the key device of integrated ultra-wideband optical systems, have become the focus of research. Meanwhile, the organic-based hybrid electro-optic modulators, which make full use of the advantages of organic electro-optic (OEO) materials (e.g. high electro-optic coefficient, fast response speed, high bandwidth, easy processing/integration and low cost) have attracted considerable attention. In this paper, we introduce a series of high-performance OEO materials that exhibit good properties in electro-optic activity and thermal stability. In addition, the recent progress of organic-based hybrid electro-optic devices is reviewed, including photonic crystal-organic hybrid (PCOH), silicon-organic hybrid (SOH) and plasmonic-organic hybrid (POH) modulators. A high-performance integrated optical platform based on OEO materials is a promising solution for growing high speeds and low power consumption in compact sizes.High performance electro-optic modulator, as the key device of integrated ultra-wideband optical systems, have become the focus of research. Meanwhile, the organic-based hybrid electro-optic modulators, which make full use of the advantages of organic electro-optic (OEO) materials (e.g. high electro-optic coefficient, fast response speed, high bandwidth, easy processing/integration and low cost) have attracted considerable attention. In this paper, we introduce a series of high-performance OEO materials that exhibit good properties in electro-optic activity and thermal stability. In addition, the recent progress of organic-based hybrid electro-optic devices is reviewed, including photonic crystal-organic hybrid (PCOH), silicon-organic hybrid (SOH) and plasmonic-organic hybrid (POH) modulators. A high-performance integrated optical platform based on OEO materials is a promising solution for growing high speeds and low power consumption in compact sizes.Introduction
Electro-optic modulators (EOMs) convert signals from the electrical to the optical domain, which are at the heart of high-capacity optical communication, broadband microwave photonics, sensing, and quantum technologies[1-4]. To fulfill the requirements of booming information technologies, such as big data, internet of things (IoT), cloud-based services, and autonomous vehicles, as well as the artificial intelligence/machine learning (AI/ML), EOMs should be able to chip-scale integrate with electronics at high-density[1,3]. However, EOMs have long suffered from the substantial size mismatch with electronic circuits together with issues of energy efficiency, bandwidth, optical loss, drive voltage, and signal distortion. Tremendous efforts have been made in the past few decades to optimize the performance of EOMs and improve the integration with electronics[1,5]. Recent advances of silicon photonics provide dense integration of complex photonic functionalities, using mature technology and process toolsets from complementary metal–oxide–semiconductor (CMOS) electronics[6–8]. Due to lack of intrinsic second-order non-linearity, silicon photonics rely on the plasma dispersion effect that derives from carrier injection by application of a voltage[4,9]. This plasma perturbs the transmission of light and thus effects modulation. Unlike Pockels modulators, plasma dispersion modulators do not have a linear dependence of voltage and refractive index change Δn (thus, they show chirp). Most recently, various heterogeneous approaches that combine Pockels-type electro-optic (EO) materials with dielectric or plasmonic waveguide structures have been explored to introduce better solutions for silicon devices[10–12].
Currently used materials for modulators include thermo-optic materials[13], III–V semiconductor (electrical absorption materials, e.g., GaAs, InP)[14], inorganic crystal materials (lithium niobite)[15], liquid crystals (LC) and organic electro-optic (OEO) materials. The process of refractive index change for thermo-optic materials and LCs is relatively slow and they cannot be used in high-speed EO modulation. Crystalline inorganic materials, which generally have low optical loss and good stability, are widely used for a long time in fiber optic networks, especially the thin-film lithium niobite on insulator (LNOI) shows the good performance[16,17]. However, due to the relatively low EO activity, the EO modulators based on lithium niobate have long propagation length and large footprints, which might be difficult to integrate with high-density. III–V semiconductors modulate light via changing the wavelength of an optical resonance caused by an applied electric field. During electro-absorption modulation, both index of refraction and absorbance change, which perhaps leads to the unwanted phenomenon of “chirp”, altering the signal waveform together with high optical loss near the half-maximum of absorption.
OEO materials are of great interest because they offer high EO coefficients, fast response speed, and extremely high bandwidth[9,18–27]. The Pockels effect of OEO materials comes from the conjugated π-electron system of nonlinear optical (NLO) molecules, which has a few femtoseconds phase relaxation time and enables the theoretical bandwidth more than 15 THz. Significant improvements in device performance have been made by silicon-organic hybrid (SOH)[28–37] and plasmonic-organic hybrid (POH)[11,38–44] Pockels effect modulators, which have been proven to be effective photonic platforms for both analog and digital applications. OEO materials generally have larger EO coefficients (300–500 pm/V), and have a potential to achieve higher EO coefficients (>1000 pm/V) based on the theoretical predictions[3,9,45]. The large EO coefficients are critical for low insertion loss, best energy efficiency, and the highest bandwidth operation. The EO coefficient r33 of OEO materials is proportional to combination of chromophore hyperpolarizability (β), electric field poling-induced acentric order of the chromophores (<cos3θ>), and chromophore number density (ρN). The half-wave voltage-length parameterVπL for an EO modulator is inversely proportional ton3r33. The past two decades of research and development have demonstrated many effective molecular engineering strategies, such as side-chain engineering, self-assembly, multi-chromophore dendrimers, site-isolation, chromophore blending, and control of chromophore shape, to increaseρN<cos3θ> (increasingρN by eliminating the inactive polymer host, while maintaining good poling efficiency)[46–59]. State-of-the-art POH modulators have already demonstrated ultra-high electro-optical bandwidths (> 500 GHz)[41], small device footprints < 10μm2, energy efficiencies ≤ 70 aJ/bit[31,42], and voltage-length parameters (VπL) of ≤ 50 V·μm with existing high-performance OEO materials[12,60]. To continually shrink theVπL values, POH and SOH modulators require novel device designs and engineering as well as improved EO activity.
This paper reviews the recent advances in OEO materials and organic-based hybrid EO modulators. To get groundbreaking performance, it is necessary to carry out synergistic innovation from rational design of OEO materials to device engineering and advancements in communication systems. Theory-guided design has been proven to be a very effective and highly efficient strategy to get high-performance chromophores. The combination of experimental and theoretical studies affords better understanding of structure-property relationships, as well as the prospects for implementation in nanoscale photonic devices. The theory-guided advances in OEO materials greatly promote the development of SOH and POH devices. In summary, the design paradigms for both materials and devices have changed dramatically over the past several years, and this review summarizes and discusses the importance of these changes.
Organic electro-optic (OEO) materials
Organic-EO materials are generally composed of two parts: the first part is the polymer that provides the substrate, which determines the refractive index and thermal properties of the material, the second part is the active chromophore that performs the EO conversion function[5].
The influencing factors of the EO performance is as shown in Eq. (1):
whereg(ω, n) is the modified Lorentz−Onsager factor taking into account the dielectric properties of the environment surrounding the chromophores.n is the refractive index of the material at the operational wavelength.
To activate the macroscopic EO effect, the organic chromophores need to be poled in the polymer network by heating them close to their glass-transition temperaturesTg to induce the alignment while applying an external electric field. An active push-pull chromophore contains a p-conjugated bridge which is end-capped with a donor and an acceptor (D-π-A). To optimize the molecular first hyperpolarizabilityβ of the EO chromophores, the electron density distribution needs to be fine-tuned through chemical modification of molecular constituents. The approaches for improving molecularβ generally include using stronger donor/acceptor combination, extending conjugating system, or low-aromatic π-bridge, and so on. With the enhancement of molecularβ, however, the molecular dipole moments rapidly increase, induce the strong anti-parallel aggregation between chromophores and make it very difficult to get acentric order under electric field poling. The chromophores with high-β need to be well-engineered with enough steric hindrance to inhibit unwanted aggregations. There are many structure-engineering strategies that have been proved effective to isolate the chromophores with each other, such as side-chain engineering, dendritic structure, and so on. Our group designed a series of high-performance chromophores with stronger electron-donors that also serve as the bulky groups to isolate with each other, as shown inFig. 1.
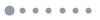
Figure 1.EO chromophores with stronger electron-donors.
Furthermore, 1,1,7,7-tetramethyl-julolidine was proved to provide not only the strong electron-donating ability but also the excellent steric hindrance in our group inFig. 1 (Chromophore representative: WJ1, A and A2)[21,22,24,51,52,59]. WJ1 was synthesized using a facile route to get a high yield (36%)[52]. The conjugating structure of the chromophore was protected by the tetramethyl groups, thiophene ring and chlorohexyloxy group, of which the steric effect and electron-donating ability enhance the molecular nonlinearity. Due to the greatly weakened dipole-dipole interactions, WJ1 can be doped in amorphous polycarbonate (APC) at a higher concentration (40 wt%) with no terrible dipole-dipole aggregations compared with most of the reported guest-host EO polymers. The bestr33 value of electric-poled WJ1/APC films is 337 pm/V, which is comparable with some outstanding materials. CLD- and FTC-types chromophores were also synthesized using 1,1,7,7-tetramethyl-julolidine as the donor. FTC-type chromophore A2 showed a relatively highr33 value of 223 pm/V in APC films by 25 wt%[21].
The chromophores using 4-(diarylamino)phenyl as donor have demonstrated significantly enhanced thermo- and photostability compared to their 4-(dialkylamino)phenyl analogues[61]. Due to the weak electron-donating ability and strongπ–π stacking interactions, however, these chromophores have poor performance in achieving larger33 in poled films. Ther33 values derived from these chromophores are lower than lithium niobate. Dalton’s group and Jen’s group have designed many chromophores with stronger 4-(diarylamino)phenyl donors by introducing alkoxyl groups into donor, or replacing the phenyl-ring by heteroaromatic rings that could increase donor strength and reduce aromaticity and ionization potential[61,62]. We designed a new electron-donor bis-(4-diarylamino)phenyl group based on bis-(4-alkoxylphenyl)phenyl group by replacing alkoxyl groups with alkylamino groups. The bis-(4-alkylamino)phenyl-amino donor exhibits stronger electron-donating ability than bis-(4-alkoxylphenyl)phenyl-amino donors. InFig. 1, the chromophore T3 showed the bestr33 value of 95 pm/V which was 1.6-fold of T2 performance[18].
A series of Y-type chromophores using Michlers ethylketone as the donor was first reported by our group, which had strong electron-donating ability and large steric hindrance, as shown inFig. 1[54,58,63–65]. The molecular conformation, including the hindrance of the donor and π-bridge, effectively isolates the chromophores and weakens the dipole–dipole interactions. Density functional theory (DFT) was used to calculate the HOMO–LUMO energy gaps and first-order hyperpolarizability of these chromophores. These chromophores showed better thermal stability with their decomposition temperatures all above 220 °C. Most importantly, the high molecular hyperpolarizability of these chromophores can be effectively translated into large EO coefficients in poled polymers due to the bulky donor structure. The poled films of FTC-yh1 and FTC-yh2 displayedr33 values of 149 and 143 pm/V at the concentration of 25 wt% in amorphous polycarbonate (APC)[63]. To improve EO activity based on FTC-yh1, two long alkoxy chains were introduced into thiophene-bride to effectively isolate with other chromophores, and using stronger electron acceptor CF3-Ph-tricyanofuran (CF3-Ph-TCF) rather than TCF can significantly improve theβ value. The poled films of 25 wt% and 35 wt% chromophore F in APC exhibited 305 and 358 pm/V respectively which were comparable with high-performance CLD-type chromophores[54].
In recent years, the research focus has been shifted from electrically poled polymer composite materials to high-number-density neat (pure) chromophore materials that yieldr33 larger than 500 pm/V in thin films[66]. Moreover, theory has led the way to utilization of OEO materials with controlled lattice dimensionality (e.g., two-dimensional or Bessel lattice materials). The dipole-dipole aggregations can be harnessed by well-engineered molecular configurations. Dalton’s group tried to do the poling for the neat JRD1 and YLD124 films that were prepared without EO inactive polymers, as shown inFig. 2[56,66]. Due to the greatly reduced electric resistance of neat chromophore films in poling, large leakage current was observed that will cause huge poling field loss. To overcome this problem, many kinds of dielectric materials have been used as charge blocking layers (CBLs) by inserting between EO layer and electrode[66,67]. By using CBLs, the poled JRD1 film exhibited greatly enhancedr33 value of 556 pm/V[66].
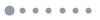
Figure 2.High performance chromophores for neat film poling.
Theoretical predictions suggest that the performance of OEO materials can be considerably greater than 1000 pm/V. Recently, significant progress has been made in theory-guided design for OEO chromophores that permits screening of many structures by evaluating their calculated hyperpolarizabilities, band gaps, and dipole moments, and finding materials optimized for multiple design criteria[68,69]. Dalton’s group designed and synthesized several new chromophores with hugely improved molecularβ andr33 values. As shown inFig. 2, BAY1 and BAH13 exhibited the maximumr33 values close to 1100 pm/V. Titanium oxide and hafnium oxide were used as the CBLs. The EO performance of BAH13 was also evaluated by POH devices in which BAH13 could greatly reduce theVπL.
To address the challenge of long-term and high-temperature stability of OEO materials, a crosslinkable EO system consisting of two chromophores, HLD1/HLD2, that can be electric field poled and then thermally crosslinked in situ has been used to form a stable system, as shown inFig. 3(a). A maximum ofr33 of 290 ± 30 pm/V was obtained in a cross-linked film and greater than 99% of the initialr33 value was maintained after heating to 85 °C for 500 h inFig. 3(b)[27].
![(Color online) (a) Chemical structure for chromophores HLD1, HLD2, cross-linker C1, and polymer P1. (b) Temporal stability of the poled films HLD1/HLD2, HLD2/C1, HLD2/P1, JRD1/APC, and JRD1/PMMA at the curing temperature of 85 °C. Reproduced with permission from Ref. [27]. Copyright 2020, American Chemical Society.](/Images/icon/loading.gif)
Figure 3.(Color online) (a) Chemical structure for chromophores HLD1, HLD2, cross-linker C1, and polymer P1. (b) Temporal stability of the poled films HLD1/HLD2, HLD2/C1, HLD2/P1, JRD1/APC, and JRD1/PMMA at the curing temperature of 85 °C. Reproduced with permission from Ref. [27]. Copyright 2020, American Chemical Society.
Though achieving very larger33 values of about 1000 pm/V in bulk thin film, the EO performance of OEO materials in POH or SOH devices is about 200 or 400 pm/V through the calculation of device’s half-wave voltage. In the future, the research will focus on how to improve the EO performance in SOH, POH devices or other type nano-photonics.
Organic-based heterogeneous hybrid electro-optic devices
Photonic crystal-organic hybrid (PCOH) modulator
Photonic crystals are a type of artificial functional material that was first proposed in the late 1980s, which are formed by periodic arrangement of dielectric materials with different dielectric constants in space. If a line defect is introduced into a photonic crystal, then a photonic crystal optical waveguide (PCW) can be generated.
In 2008, Brosiet al. proposed a slot-photonic crystal slow-light phase modulator with a length of only 80μm, a bandwidth of 78 GHz and a transmission speed of 100 Gbit/s[70]. As shown inFig. 4(a), the slow-light phase modulator consists of slotted photonic crystal waveguides (150 nm) filled with nonlinear OEO materials which form the interaction region. Due to the slow-light effect of the slot-photonic crystal waveguide, the velocity of the light field group in the modulation region decreased, the interaction time between the light field and the microwave electric field is significantly increased, and then the length of the modulator is reduced. In 2010, Chen’s group demonstrated a PCOH modulator integrating silicon photonic crystal (PC) slot waveguide (75 nm) and AJ-CKL1/APC electro-optic polymer. An ultra-efficient EO modulation based on a half-wave voltage length productVπL = 0.56 V·mm and an effective electro-optic coefficientr33 = 132 pm/V is obtained by using slow-light effect and strong field constraints in a slot waveguide[71]. A new EO polymer-filled silicon slot PC waveguide modulator was reported and the structural diagram is shown inFig. 4(b). The half-wave voltageVπ of the PCOH modulator is 0.97 V, and the half-wave voltage length productVπL is 0.291 V·mm and then the 3dB bandwidth is raised to 15 GHz in 2016[72,73].
![(Color online) (a) Schematic of the slot-photonic crystal slow-light phase modulator and dominant electric field component Ex at quasi-TE mode. Reprinted with permission from Ref. [70], Copyright 2008, The Optical Society. (b) Scanning electron microscopy (SEM) images of the fabricated device. Reprinted with permission from Ref. [73], Copyright 2016, The Optical Society.](/Images/icon/loading.gif)
Figure 4.(Color online) (a) Schematic of the slot-photonic crystal slow-light phase modulator and dominant electric field component Ex at quasi-TE mode. Reprinted with permission from Ref. [70], Copyright 2008, The Optical Society. (b) Scanning electron microscopy (SEM) images of the fabricated device. Reprinted with permission from Ref. [73], Copyright 2016, The Optical Society.
Silicon-organic hybrid (SOH) modulators
Silicon photonics offers great potential for its CMOS compatibility with electronics and low-cost optoelectronic integration technologies. In recent years, various silicon-based active and passive optical waveguide devices have developed rapidly, and silicon photonics is a promising platform for highly integrated photonic chips due to its high-index-contrast and the compatibility with the mature complementary. However, silicon has nearly no electro-optic effect, so the traditional silicon modulators mainly rely on free carrier dispersion effect to achieve electro-optic modulation. Therefore, it is difficult for silicon modulators to achieve 100 GHz bandwidth and eliminate the nonlinear response[17].
Integrating the excellent electro-optic materials into a mature silicon photonics platform is an effective means to overcome bandwidth and loss limits that stem from the physics of the plasma dispersion effect. SOH modulator combines with silicon-on-insulator (SOI) waveguides and the functional organic nonlinear materials with larger33 to realize the large bandwidth and low half-wave voltage ultra-compact modulator. Around 2011, a SOH phase modulator was first demonstrated by the Christian Koos and Juerg Leuthold's group at a data rate of 42.7 Gbit/s with a widely open eye diagrams[74]. In the SOH device, the silicon slot waveguide by increasing the doping concentration of the silicon strips can realize low resistivity, and the EO bandwidth is increased according to the corresponding RC time constant. As shown inFig. 5(a), the electro-optic polymer M1 consists of chromophores dispersed in APC with the electro-optic coefficient of 70 pm/V, and the slot width of 120 nm and the 1.7 mm long phase shifting section is adopted in the device with aVπ of 5.3 V. The SOH phase modulator was reported by the same group with the frequency response of more than 100 GHz by using a device as short as 500μm, a highly conductive electron accumulation layer and an improved gate insulator inFig. 5(b)[10]. The results indicate that the RC time constant is not a speed limitation of the device at 100 GHz.
![(Color online) (a) The SOH phase modulator with an SiO2 film on top of the silicon strips which cover with the gate electrode. Reproduced with permission from Ref. [74]. Copyright 2011, Optical Society of America. (b) 100 GHz SOH phase modulator. Reproduced with permission from Ref. [10]. Copyright 2014, Nature Publishing Group. (c) Ultra-low half-wave voltage of 0.21 V SOH MZM. Reproduced with permission from Ref. [34]. Copyright 2018, Optical Society of America. (d) Capacitivity coupled SOH MZM with high-κ slotlines. Reproduced with permission from Ref.[75]. Copyright 2021, Optical Society of America. (e) High-temperature-resistant SOH MZM working up to 200 Gbit/s over 100 °C. Reproduced with permission from Ref. [77]. Copyright 2020, Nature Publishing Group. (f) The structure of SOH MZM by optimizing the strip-to-slot mode converter. Reproduced with permission from Ref. [78]. Copyright 2020, Optics and Precision Engineering.](/Images/icon/loading.gif)
Figure 5.(Color online) (a) The SOH phase modulator with an SiO2 film on top of the silicon strips which cover with the gate electrode. Reproduced with permission from Ref. [74]. Copyright 2011, Optical Society of America. (b) 100 GHz SOH phase modulator. Reproduced with permission from Ref. [10]. Copyright 2014, Nature Publishing Group. (c) Ultra-low half-wave voltage of 0.21 V SOH MZM. Reproduced with permission from Ref. [34]. Copyright 2018, Optical Society of America. (d) Capacitivity coupled SOH MZM with high-κ slotlines. Reproduced with permission from Ref.[75]. Copyright 2021, Optical Society of America. (e) High-temperature-resistant SOH MZM working up to 200 Gbit/s over 100 °C. Reproduced with permission from Ref. [77]. Copyright 2020, Nature Publishing Group. (f) The structure of SOH MZM by optimizing the strip-to-slot mode converter. Reproduced with permission from Ref. [78]. Copyright 2020, Optics and Precision Engineering.
Low energy consumption is a problem that must be considered. In 2015, the power consumption of a 1-mm-long SOH modulator was reduced to 0.7 fJ/bit, and the device was able to be operated at data rates of up to 40 Gbit/s[31]. In Ref. [34], a SOH modulator with very low half-wave voltage (0.21 V) in a 1.5-mm-long device was reported (Fig. 5(c)). Organic chromophore JRD1 as a cladding, and the maximumn3r33 amounts to 2300 pm/V withr33 of 390 pm/V was achieved in the SOH device. To overcome the RC limitation of SOH modulator, BaTiO3 with high-κ can avoid the resistive slab and form a large coupling capacitor between the optical slot waveguides. A bandwidth of 76 GHz and aVπ of 1.3 V are achieved in the 1-mm-long SOH capacitively coupled modulator (Fig. 5(d))[75].
The reported results showed that the poled polymer film can still maintain good electro-optic activity in the cryogenic region of 7 K[76]. However, the thermal stability and long-term reliability of OEO materials have always been concerned because thermal relaxation of chromophores will happen when its working and storage temperature are higher thanTg. It illustrates that the thermal stability of the OEO materials seems to have been solved in the following work. As shown in theFig. 5(e), the SOH modulator combined an ultra-highTgof 172 °C EO polymer with silicon rib waveguides. The modulator showed 200 Gbit/s PAM4 modulations, and feature excellent reliability at ambient temperatures up to 110 °C[77].
Silicon slot waveguides filled with OEO polymers (r33 = 150 pm/V) in a SOH Mach Zehnder modulator (MZM) were reported in internal research group. According to the simulation results,VπL of 0.45 V·mm and bandwidth of 70 GHz could be achieved[78]. The overall structure and the cross-section structure of the modulator are shown inFig. 5(f). The strip-to-slot mode converter has a cone-shaped coupling efficiency of 99.5%, which greatly reduces the loss caused by mode conversion.
Plasmonic−organic hybrid (POH) modulators
Highly compact nanometer optoelectronic integrated chips require smaller device sizes. However, the mode size of traditional dielectric waveguide is more than half of its wavelength length due to the diffraction limit. Surface plasmon photonics are a means of providing ultra-compact and high-speed components. Surface plasmon polariton (SPP) devices have developed rapidly, such as SPPs waveguide, SPPs modulator, SPPs sensor and so on. Plasmonic−organic hybrid modulators have attracted much attention in recent years which combine many advantages of the large RF bandwidth of a travelling wave modulator, the compactness of a photonic-crystal modulator, and the energy efficiency of a ring modulator.
The size variation of the various organic-based hybrid modulators (all-organic, SOH, and POH) is shown inFig. 6(a)[1]. All-organic thin film OEO devices consist of upper and lower cladding layer and core OEO layer which are all made of polymer. The three layers are sandwiched between top and bottom electrode. To achieve low half-wave voltage, devices of 1–2 cm in length are typically required, because the electrode separations of greater than 7μm (thickness of three-layer films)[79]. Although all-organic devices achieved significant drive voltage and bandwidth, they still suffered from a large footprint for integration of electronics and photonics. As shown inFig. 6(b), in the SOH modulator, the electrical field drops only across the silicon slot waveguide filled with the OEO materials, and this allows strong overlap of optical and RF electric fields, reducing device length to a few hundred micrometers[79]. POH modulator uses metal-slot waveguides rather than silicon slot waveguides and exploits stronger confinement of light for surface plasmon polaritons, thereby reducing device lengths to a few tens of micrometers and electrode spacing to less than 50 nm. For the 2 V CMOS drive, the phase shifter lengths for lithium niobate and Si PN junction are 1 cm and 2 mm, while phase shifter lengths for SOH and POH are only 200 and 25μm, respectively. Therefore, the SOH and POH showed the small footprint for the CMOS process-compatible chip-scale integration.VπL values from 40 to 50 V·μm have been reported (symbols) in JRD1 and DLD164 for POH devices inFig. 6(c), and even lower values based on demonstrated improvements in new materials[69].
![(Color online) (a) The relative size of all-organic, SOH and POH modulator. Reproduced with permission from Ref. [1]. Copyright 2017, American Chemical Society. (b) Variation of halfwave voltage (Vπ) with electrode length/device length (L) for various types of devices. Reproduced with permission from Ref. [79]. Copyright 2021, American Chemical Society. (c) Comparison measured (symbols) and computationally predicted (lines)VπL values for JRD1, DLD164, and BAH13 organic OEO materials at 1550 nm in a POH MZM. Reproduced with permission from Ref. [69]. Copyright 2022, Royal Society of Chemistry.](/Images/icon/loading.gif)
Figure 6.(Color online) (a) The relative size of all-organic, SOH and POH modulator. Reproduced with permission from Ref. [1]. Copyright 2017, American Chemical Society. (b) Variation of halfwave voltage (Vπ) with electrode length/device length (L) for various types of devices. Reproduced with permission from Ref. [79]. Copyright 2021, American Chemical Society. (c) Comparison measured (symbols) and computationally predicted (lines)VπL values for JRD1, DLD164, and BAH13 organic OEO materials at 1550 nm in a POH MZM. Reproduced with permission from Ref. [69]. Copyright 2022, Royal Society of Chemistry.
In 2014, J. Leuthold's group first reported a new POH phase modulator, and the structure diagram is shown inFig. 7(a)[11]. Light enters the plasmon waveguide from the silicon waveguide and propagates in the surface plasmon (SPP) mode. The gap is filled with OEO materials, and the phase modulation is realized by applying voltage to the metal. The device is only 29μm long, operates up to 40 Gbit/s, and has a frequency response of at least 65 GHz. Next, a POH intensity MZM was demonstrated with a bandwidth of 70 GHz in 2015, and the scanning electron microscope image of the MZM component is shown inFig. 7(b). The device has aVπL of 0.06 V·mm and an energy consumption of 25 fJ/bit, which is attributed to the filling of the slot with a polymer called DLD-164[12].
![(Color online) (a) A high-speed POH phase modulator designed and fabricated. Reproduced with permission from Ref. [11]. Copyright 2014, Nature Publishing Group. (b) POH MZM with metal-insulator-metal plasmonic slot waveguide. Reproduced with permission from Ref. [12]. Copyright 2015, Nature Publishing Group. (c) All-plasmonic MZM using a single metal layer without the silicon waveguide. Reproduced with permission from Ref. [38]. Copyright 2017, Nature Publishing Group. (d) Low-loss plasmonic electro-optic ring modulator. Reproduced with permission from Ref. [40]. Copyright 2018, Nature Publishing Group.](/Images/icon/loading.gif)
Figure 7.(Color online) (a) A high-speed POH phase modulator designed and fabricated. Reproduced with permission from Ref. [11]. Copyright 2014, Nature Publishing Group. (b) POH MZM with metal-insulator-metal plasmonic slot waveguide. Reproduced with permission from Ref. [12]. Copyright 2015, Nature Publishing Group. (c) All-plasmonic MZM using a single metal layer without the silicon waveguide. Reproduced with permission from Ref. [38]. Copyright 2017, Nature Publishing Group. (d) Low-loss plasmonic electro-optic ring modulator. Reproduced with permission from Ref. [40]. Copyright 2018, Nature Publishing Group.
In contrast to the previous two works, the all-plasmonic MZM without any silicon waveguide is reported inFig. 7(c)[38]. All the elements (grating couplers, splitters, polarization rotators and active section) are included in a single metal layer in this POH MZM. The length of phase shifter is 16μm, and the half-wave voltage is 10 V. The frequency response of the device exhibits no speed limitation up to 70 GHz and 116 Gbit/s PAMs modulation formats.
The electron motion generates heat through ohmic losses for plasmonics, which has led to a broad view that plasmonics is too lossy for optical transmission. To reduce the intertion loss of POH modulator, a plasmonic electro-optic ring modulator was reported in theFig. 7(d). The ohmic losses can be bypassed by using “resonant switching”. The intertion loss of the device is only 2.5 dB which can be reduced by more than 6 dB relative to non-resonant MZM device. The bandwidth of the plasmonic resonator is beyond 110 GHz[40].
Plasmonics is indeed a viable path to an ultracompact, highest-speed, and low-cost technology modulator that can be used in a wide range of fields. The applications of POH modultors have been carried out. Terahertz wireless applications demands modulators with frequency responsed to the sub-terahertz range, high power handing, and very low nonlinear distortions. Beyond 500 GHz, Vπ of 3 V, and high linearity 25μm-long POH modulator was experimentally demonstrated, which can achieve a 222 GBd on-off-keying transmitter in theFigs. 8(a) and8(b)[41,80]. The POH modulator shows good performance, not only in the digital signals but also in the sub-terahertz radio-over-fiber analog optical link. The POH modulator has high linearity with a third-order input intercept point (IIP3) of 18.9 dBm. Meanwhile, an analog RoF link over 100 GHz bandwidth (220–325 GHz) was used to prove the capability of direct terahertz-to-optical-to-terahertz conversion of the POH modulator, and the experiment represents the link has a flat response over the complete 220–325 GHz frequency range. Based on these results, POH modulators have potential applications in the general field of microwave photonics, 5G wireless communications, antenna remoting, internet of things, sensing and more analogy links for the sub-terahertz range.
![(Color online) (a) Beyond 500 GHz POH MZM used for sub-THz microwave photonics. Reproduced with permission from Ref. [41]. Copyright 2019. American Institute of Physics. (b) 222 GBd on-off-keying transmitter based on POH MZM. Reproduced with permission from Ref. [80]. Copyright 2020. Optical Society of America. (c) Compact IQ electro-optic modulator operated with sub-1-V driving electronics. Reproduced with permission from Ref. [42]. Copyright 2019. Nature Publishing Group. (d) Symbol rates 100 GBd monolithically integrated electro-optical transmitter based on POH MZM. Reproduced with permission from Ref. [44]. Copyright 2020. Nature Publishing Group.](/Images/icon/loading.gif)
Figure 8.(Color online) (a) Beyond 500 GHz POH MZM used for sub-THz microwave photonics. Reproduced with permission from Ref. [41]. Copyright 2019. American Institute of Physics. (b) 222 GBd on-off-keying transmitter based on POH MZM. Reproduced with permission from Ref. [80]. Copyright 2020. Optical Society of America. (c) Compact IQ electro-optic modulator operated with sub-1-V driving electronics. Reproduced with permission from Ref. [42]. Copyright 2019. Nature Publishing Group. (d) Symbol rates 100 GBd monolithically integrated electro-optical transmitter based on POH MZM. Reproduced with permission from Ref. [44]. Copyright 2020. Nature Publishing Group.
Heniet al. demonstrated an in-phase/quadrature (IQ) POH modulators, and the active region was 4 × 25µm × 3µm with sub-1-V driving electronics. As shown inFig. 8(c), the devices exhibit low electrical energy consumptions of 0.07 fJ/bit at 50 Gbit/s, 0.3 fJ/bit at 200 Gbit/s, and 2 fJ/bit at 400 Gbit/s with organic chromophore HD-BB-OH/YLD124 filling the slot[42]. The IQ POH modulator can be used in long-haul and short-haul communications.
Recently, a symbolic rate over 100 GBd single-chip electro-optical transmitter that combines advanced bipolar CMOS with silicon plasmonic was reported. This is a great progress, because it addresses key challenges in single-chip integration through the co-design of electronics and plasmonic layers in theFig. 8(d)[44]. The HLD1/HLD2 organic material in the plasmonic modulator shows very excellent electro-optic and thermal-stability performance. The POH modulator based on the above organic materials can operate stable data modulation at temperatures above 112 °C.
The calculated capacitance of POH modulator is approximately several fF, so the RC bandwidth can break through the limitation of RC time constant and be much greater than 1 THz. Meanwhile, the Pockels effect is based on the reorganization of π-electrons within a nanometer-long molecule of OEO materials and is estimated to be on the timescale of femtoseconds or attoseconds. The Pockels bandwidth will be much greater than 1 THz. Therefore, the POH modulator has a great space for the ultra-large bandwidth.
Conclusion
The recent developments of OEO materials and organic-based hybrid modulators were reviewed. The outstanding characteristic of OEO materials is their design flexibility which makes OEO materials have high electro-optic activity, fast response speed, high processability, good thermal stability and low cost. Meanwhile, the OEO material is flexible and compatible with many processes (CMOS, silicon photonics) without bonding. The defect for both datacom and telecom system is the lack of performance, specifically as it relates to very high speeds and very low power consumption. Heterogeneous integration based on the organic materials is a very promising solution for high-speed optical modulators and communication system.
Table 1 presents various performance for the high-speed modulators with operating principle of Pockels effect in SOH, POH, PCOH, LNOI, and LN/Si (LN film bonded to SOI platform). The linear Pockels effect can offer intrinsically good linearity. SOH has the lowest half-wave voltage (0.21 V) because of the large EO coefficient (r33 = 390 pm/V) of OEO polymer in the device. The bandwidth of SOH is intermediate between POH and thin film lithium niobite based modulators. POH modulators have the highest bandwidth and the smallest footprint, which make them promising materials for ultra-high speed and low energy consumption integrated system on a chip (SOC). Compared with the LNOI, POH modulators show the large propagation losses which can be solved through waveguide structure and manufacturing process[40]. PCOH modulators are less studied, perhaps because they need to fabricate complex photonic crystals. The LNOI modulator exhibits ultra-low propagation losses, but may be difficult to achieve low driving voltage for CMOS-level and small size simultaneously owing to the fixedr33.
Table Infomation Is Not Enable
It is reasonable to find suitable applications for different materials because discrete device applications will likely be based on low optical loss materials such as lithium niobite and silicon. For the application of chip-scale integration of electronics and photonics, the requirement of small size close toμm and CMOS-level driving voltage less than 1 V will likely favor OEO materials. OEO materials are suitable for the satellite and space telecommunications because they may be used to develop small size, lightweight, excellent efficiency and radiation resistance devices[79]. The range of applications for electro-optics is broad, besides the high-capacity telecommunications and ultra-bandwidth microwave photonics, electro-optics can be used in Lidar, sensor, computing, THz and other areas.
In the future, new OEO materials can be designed and synthesized to optimize the EO performance, refractive index, optical loss and EO thermal-stability, and offer many potential possibilities for high-performance EO modulators. SOH modulator shows lowVπL and energy consumption, and the aim is to improve the bandwidth and maturity of processing technology. A POH device is a highly efficient modulator to achieve unprecedented performance (very high speed, low power consumption) and compactness, and designing the new waveguide structure and optimizing preparation process should be attracted attention to reduce optical loss. More and more research institutions, universities and companies (e.g., Lightwave Logic) have already studied the organic EO materials devices, and high-performance optical circuits and system could be expected.
Acknowledgements
We are grateful to the National Natural Science Foundation of China (No. 62175267), the Beijing Municipal Natural Science Foundation (No. 4192061), the Fundamental Research Funds for the Central Universities (2020MDJC13) and the Beijing Talents Foundation (2018000021223ZK45) for the financial support.
References
[1] W Heni, Y Kutuvantavida, C Haffner et al. Silicon-organic and plasmonic-organic hybrid photonics. ACS Photonics, 4, 1576(2017).
[2] I C Benea-Chelmus, T Q Zhu, F F Settembrini et al. Three-dimensional phase modulator at telecom wavelength acting as a terahertz detector with an electro-optic bandwidth of 1.25 terahertz. ACS Photonics, 5, 1398(2018).
[3] B H Robinson, L E Johnson, D L Elder et al. Optimization of plasmonic-organic hybrid electro-optics. J Lightwave Technol, 36, 5036(2018).
[4] A Rahim, A Hermans, B Wohlfeil et al. Taking silicon photonics modulators to a higher performance level: State-of-the-art and a review of new technologies. Adv Photonics, 3, 024003(2021).
[5] L R Dalton, B H Robinson, D L Elder et al. Hybrid electro-optics and chipscale integration of electronics and photonics. Proc SPIE, 10364(2017).
[6] J Wang, Y Long. On-chip silicon photonic signaling and processing: A review. Sci Bull, 63, 1267(2018).
[7] P Dong, Y K Chen, G H Duan et al. Silicon photonic devices and integrated circuits. Nanophotonics, 3, 215(2014).
[8] Y H Dong, Y Zhang, J Shen et al. Silicon-integrated high-speed mode and polarization switch-and-selector. J Semicond, 43, 022301(2022).
[9] L R Dalton, P A Sullivan, D H Bale. Electric field poled organic electro-optic materials: State of the art and future prospects. Chem Rev, 110, 25(2010).
[10] L Alloatti, R Palmer, S Diebold et al. 100 GHz silicon–organic hybrid modulator. Light Sci Appl, 3, e173(2014).
[11] A Melikyan, L Alloatti, A Muslija et al. High-speed plasmonic phase modulators. Nat Photonics, 8, 229(2014).
[12] C Haffner, W Heni, Y Fedoryshyn et al. All-plasmonic Mach-Zehnder modulator enabling optical high-speed communication at the microscale. Nat Photonics, 9, 525(2015).
[13] K Liang, C Wang, B Y Wu et al. Polymeric thermo-optic digital optical switches. Chin J Semicond, 27, 747(2006).
[14] D P Liu, J Tang, Y Meng et al. Ultra-low Vpp and high-modulation-depth InP-based electro-optic microring modulator. J Semicond, 42, 082301(2021).
[15] M B He, M Y Xu, Y X Ren et al. High-performance hybrid silicon and lithium niobate Mach–Zehnder modulators for 100 Gbit s−1 and beyond. Nat Photonics, 13, 359(2019).
[16] S Yuan, C R Hu, A Pan et al. Photonic devices based on thin-film lithium niobate on insulator. J Semicond, 42, 041304(2021).
[17] M Y Xu, X L Cai. Advances in integrated ultra-wideband electro-optic modulators. Opt Express, 30, 7253(2022).
[18] Y H Yang, F G Liu, H R Wang et al. Enhanced electro-optic activity from the triarylaminophenyl-based chromophores by introducing heteroatoms to the donor. J Mater Chem C, 3, 5297(2015).
[19] H Zhang, Y X Tian, S H Bo et al. A study on regulating the conjugate position of NLO chromophores for reducing the dipole moment and enhancing the electro-optic activities of organic materials. J Mater Chem C, 8, 1380(2020).
[20] Z A Li, H Kim, S H Chi et al. Effects of counterions with multiple charges on the linear and nonlinear optical properties of polymethine salts. Chem Mater, 28, 3115(2016).
[21] F G Liu, H J Xu, H Zhang et al. Synthesis of julolidine-containing nonlinear optical chromophores: Achieving excellent electro-optic activity by optimizing the bridges and acceptors. Dyes Pigments, 134, 358(2016).
[22] H Zhang, F Y Huo, F G Liu et al. Synthesis and characterization of two novel second-order nonlinear optical chromophores based on julolidine donors with excellent electro-optic activity. RSC Adv, 6, 99743(2016).
[23] F G Liu, H Zhang, H Y Xiao et al. Structure-function relationship exploration for enhanced electro-optic activity in isophorone-based organic NLO chromophores. Dyes Pigments, 157, 55(2018).
[24] C L Hu, F G Liu, H Zhang et al. Synthesis of novel nonlinear optical chromophores: Achieving excellent electro-optic activity by introducing benzene derivative isolation groups into the bridge. J Mater Chem C, 3, 11595(2015).
[25] F G Liu, M L Zhang, H Y Xiao et al. Auxiliary donor for tetrahydroquinoline-containing nonlinear optical chromophores: Enhanced electro-optical activity and thermal stability. J Mater Chem C, 3, 9283(2015).
[26] J Y Wu, Z Li, J D Luo et al. High-performance organic second- and third-order nonlinear optical materials for ultrafast information processing. J Mater Chem C, 8, 15009(2020).
[27] H J Xu, F G Liu, D L Elder et al. Ultrahigh electro-optic coefficients, high index of refraction, and long-term stability from Diels-alder cross-linkable binary molecular glasses. Chem Mater, 32, 1408(2020).
[28] A Honardoost, R Safian, M Teng et al. Ultralow-power polymer electro-optic integrated modulators. J Semicond, 40, 070401(2019).
[29] J D Witmer, T P McKenna, P Arrangoiz-Arriola et al. A silicon-organic hybrid platform for quantum microwave-to-optical transduction. Quantum Sci Technol, 5, 034004(2020).
[30] C Weimann, P C Schindler, R Palmer et al. Silicon-organic hybrid (SOH) frequency comb sources for terabit/s data transmission. Opt Express, 22, 3629(2014).
[31] S Koeber, R Palmer, M Lauermann et al. Femtojoule electro-optic modulation using a silicon–organic hybrid device. Light Sci Appl, 4, e255(2015).
[32] M Lauermann, S Wolf, P C Schindler et al. 40 GBd 16QAM signaling at 160 gb/s in a silicon-organic hybrid modulator. J Lightwave Technol, 33, 1210(2015).
[33] C Koos, J Leuthold, W Freude et al. Silicon-organic hybrid (SOH) and plasmonic-organic hybrid (POH) integration. J Lightwave Technol, 34, 256(2016).
[34] C Kieninger, Y Kutuvantavida, D L Elder et al. Ultra-high electro-optic activity demonstrated in a silicon-organic hybrid modulator. Optica, 5, 739(2018).
[35] C Kieninger, Y Kutuvantavida, H Miura et al. Demonstration of long-term thermally stable silicon-organic hybrid modulators at 85 °C. Opt Express, 26, 27955(2018).
[36] S Wolf, H Zwickel, W Hartmann et al. Silicon-organic hybrid (SOH) Mach-Zehnder modulators for 100 Gbit/s on-off keying. Sci Rep, 8, 2598(2018).
[37] C Kieninger, C Füllner, H Zwickel et al. SOH Mach-Zehnder modulators for 100 GBd PAM4 signaling with sub-1 dB phase-shifter loss. 2020 Opt Fiber Commun Conf Exhib OFC, 1(2020).
[38] M Ayata, Y Fedoryshyn, W Heni et al. High-speed plasmonic modulator in a single metal layer. Science, 358, 630(2017).
[39] C Hoessbacher, A Josten, B Baeuerle et al. Plasmonic modulator with >170 GHz bandwidth demonstrated at 100 GBd NRZ. Opt Express, 25, 1762(2017).
[40] C Haffner, D Chelladurai, Y Fedoryshyn et al. Low-loss plasmon-assisted electro-optic modulator. Nature, 556, 483(2018).
[41] M Burla, C Hoessbacher, W Heni et al. 500 GHz plasmonic Mach-Zehnder modulator enabling sub-THz microwave photonics. APL Photonics, 4, 056106(2019).
[42] W Heni, Y Fedoryshyn, B Baeuerle et al. Plasmonic IQ modulators with attojoule per bit electrical energy consumption. Nat Commun, 10, 1694(2019).
[43] B Baeuerle, C Hoessbacher, W Heni et al. 100 GBd IM/DD transmission over 14 km SMF in the C-band enabled by a plasmonic SSB MZM. Opt Express, 28, 8601(2020).
[44] U Koch, C Uhl, H Hettrich et al. A monolithic bipolar CMOS electronic-plasmonic high-speed transmitter. Nat Electron, 3, 338(2020).
[45] L R Dalton. Theory-inspired development of organic electro-optic materials. Thin Solid Films, 518, 428(2009).
[46] P A Sullivan, H Rommel, Y Liao et al. Theory-guided design and synthesis of multichromophore dendrimers: An analysis of the electro-optic effect. J Am Chem Soc, 129, 7523(2007).
[47] J L Liu, S H Bo, X H Liu et al. Enhanced poling efficiency in rigid-flexible dendritic nonlinear optical chromophores. J Incl Phenom Macrocycl Chem, 68, 253(2010).
[48] Z A Li, W B Wu, Q Q Li et al. High-generation second-order nonlinear optical (NLO) dendrimers: Convenient synthesis by click chemistry and the increasing trend of NLO effects. Angew Chem Int Ed Engl, 49, 2763(2010).
[49] W B Wu, J G Qin, Z Li. New design strategies for second-order nonlinear optical polymers and dendrimers. Polymer, 54, 4351(2013).
[50] S R Hammond, J Sinness, S Dubbury et al. Molecular engineering of nanoscale order in organic electro-optic glasses. J Mater Chem, 22, 6752(2012).
[51] Z Chen, A R Zhang, H Y Xiao et al. Tailoring the chemical structures and nonliear optical properties of julolidinyl-based chromophores by molecular engineering. Dyes Pigments, 173, 107876(2020).
[52] J Y Wu, S H Bo, J L Liu et al. Synthesis of novel nonlinear optical chromophore to achieve ultrahigh electro-optic activity. Chem Commun, 48, 9637(2012).
[53] X H Zhou, J D Luo, J A Davies et al. Push-pull tetraene chromophores derived from dialkylaminophenyl, tetrahydroquinolinyl and julolidinyl moieties: Optimization of second-order optical nonlinearity by fine-tuning the strength of electron-donating groups. J Mater Chem, 22, 16390(2012).
[54] S H Bo, Y Li, T T Liu et al. Systematic study on the optimization of a bis(N, N-diethyl)aniline based NLO chromophorevia a stronger electron acceptor, extended π-conjugation and isolation groups. J Mater Chem C, 10, 3343(2022).
[55] D L Elder, S J Benight, J S Song et al. Matrix-assisted poling of monolithic bridge-disubstituted organic NLO chromophores. Chem Mater, 26, 872(2014).
[56] W W Jin, P V Johnston, D L Elder et al. Structure-function relationship exploration for enhanced thermal stability and electro-optic activity in monolithic organic NLO chromophores. J Mater Chem C, 4, 3119(2016).
[57] D L Elder, C Haffner, W Heni et al. Effect of rigid bridge-protection units, quadrupolar interactions, and blending in organic electro-optic chromophores. Chem Mater, 29, 6457(2017).
[58] H Zhang, Y H Yang, H Y Xiao et al. Enhancement of electro-optic properties of bis(N, N-diethyl)aniline based second order nonlinear chromophores by introducing a stronger electron acceptor and modifying the π-bridge. J Mater Chem C, 5, 6704(2017).
[59] A R Zhang, H Y Xiao, C C Peng et al. Microwave-assisted synthesis of novel julolidinyl-based nonlinear optical chromophores with enhanced electro-optic activity. RSC Adv, 4, 65088(2014).
[60] S Ummethala, T Harter, K Koehnle et al. THz-to-optical conversion in wireless communications using an ultra-broadband plasmonic modulator. Nat Photonics, 13, 519(2019).
[61] Y J Cheng, J D Luo, S Hau et al. Large electro-optic activity and enhanced thermal stability from diarylaminophenyl-containing high-β nonlinear optical chromophores. Chem Mater, 19, 1154(2007).
[62] J A Davies, A Elangovan, P A Sullivan et al. Rational enhancement of second-order nonlinearity: Bis-(4-methoxyphenyl) hetero-aryl-amino donor-based chromophores: Design, synthesis, and electrooptic activity. J Am Chem Soc, 130, 10565(2008).
[63] Y H Yang, H J Xu, F G Liu et al. Synthesis and optical nonlinear property of Y-type chromophores based on double-donor structures with excellent electro-optic activity. J Mater Chem C, 2, 5124(2014).
[64] Y H Yang, J L Liu, M L Zhang et al. The important role of the location of the alkoxy group on the thiophene ring in designing efficient organic nonlinear optical materials based on double-donor chromophores. J Mater Chem C, 3, 3913(2015).
[65] Y H Yang, H R Wang, F G Liu et al. The synthesis of new double-donor chromophores with excellent electro-optic activity by introducing modified bridges. Phys Chem Chem Phys, 17, 5776(2015).
[66] W W Jin, P V Johnston, D L Elder et al. Benzocyclobutene barrier layer for suppressing conductance in nonlinear optical devices during electric field poling. Appl Phys Lett, 104, 243304(2014).
[67] S Huang, T D Kim, J D Luo et al. Highly efficient electro-optic polymers through improved poling using a thin TiO2-modified transparent electrode. Appl Phys Lett, 96, 243311(2010).
[68] H J Xu, D L Elder, L E Johnson et al. Electro-optic activity in excess of 1000 pm V–1 achieved via theory-guided organic chromophore design. Adv Mater, 33, 2104174(2021).
[69] H J Xu, D L Elder, L E Johnson et al. Design and synthesis of chromophores with enhanced electro-optic activities in both bulk and plasmonic-organic hybrid devices. Mater Horiz, 9, 261(2022).
[70] J M Brosi, C Koos, L C Andreani et al. High-speed low-voltage electro-optic modulator with a polymer-infiltrated silicon photonic crystal waveguide. Opt Express, 16, 4177(2008).
[71] C Y Lin, X L Wang, S Chakravarty et al. Electro-optic polymer infiltrated silicon photonic crystal slot waveguide modulator with 23 dB slow light enhancement. Appl Phys Lett, 97, 093304(2010).
[72] X Y Zhang, A Hosseini, S Chakravarty et al. Wide optical spectrum range, subvolt, compact modulator based on an electro-optic polymer refilled silicon slot photonic crystal waveguide. Opt Lett, 38, 4931(2013).
[73] X Y Zhang, C J Chung, A Hosseini et al. High performance optical modulator based on electro-optic polymer filled silicon slot photonic crystal waveguide. J Lightwave Technol, 34, 2941(2016).
[74] L Alloatti, D Korn, R Palmer et al. 42.7 Gbit/s electro-optic modulator in silicon technology. Opt Express, 19, 11841(2011).
[75] S Ummethala, J N Kemal, A S Alam et al. Hybrid electro-optic modulator combining silicon photonic slot waveguides with high-k radio-frequency slotlines. Optica, 8, 511(2021).
[76] D H Park, V Yun, J Luo et al. EO polymer at cryogenic temperatures. Electron Lett, 52, 1703(2016).
[77] G W Lu, J X Hong, F Qiu et al. High-temperature-resistant silicon-polymer hybrid modulator operating at up to 200 Gbit s−1 for energy-efficient datacentres and harsh-environment applications. Nat Commun, 11, 4224(2020).
[78] Y H Zou, Y M Wang, X X Zhang et al. Optimal design and preparation of silicon-organic hybrid integrated electro-optic modulator. Opt Precision Eng, 28, 2138(2020).
[79] D L Elder, L R Dalton. Organic electro-optics and optical rectification: From mesoscale to nanoscale hybrid devices and chip-scale integration of electronics and photonics. Ind Eng Chem Res, 61, 1207(2022).
[80] W Heni, B Baeuerle, H Mardoyan et al. Ultra-high-speed 2: 1 digital selector and plasmonic modulator IM/DD transmitter operating at 222 GBaud for intra-datacenter applications. J Lightwave Technol, 38, 2734(2020).
[81] C Wang, M Zhang, X Chen et al. Integrated lithium niobate electro-optic modulators operating at CMOS-compatible voltages. Nature, 562, 101(2018).
[82] P O Weigel, J Zhao, K Fang et al. Bonded thin film lithium niobate modulator on a silicon photonics platform exceeding 100 GHz 3-dB electrical modulation bandwidth. Opt Express, 26, 23728(2018).
[83] H Zwickel, J N Kemal, C Kieninger et al. Electrically packaged silicon-organic hybrid (SOH) I/Q-modulator for 64 GBd operation. Opt Express, 26, 34580(2018).