Author Affiliations
1Department of Electronic Engineering, East China Normal University, Shanghai 200241, China2Ministry of Education Nanophotonics & Advanced Instrument Engineering Research Center, East China Normal University, Shanghai 200241, China3Shanghai Institute of Intelligent Electronics & Systems, Fudan University, Shanghai 200433, China4Institute for Solid State Physics, The University of Tokyo, 5-1-5 Kashiwanoha, Kashiwa, Chiba 277-8581, Japan5e-mail: egweng@ee.ecnu.edu.cn6e-mail: sqchen@ee.ecnu.edu.cnshow less
Fig. 1. Preparation of CsPbX3 single microcrystals. (a) Illustration of the synthesis of CsPbX3 microcrystals by the liquid-phase method. First, drop a certain amount of DMF–CsPbX3 solution onto the substrate, which was preheated to a given temperature Th, and keep the temperature unchanged in ambient air. Then, evaporate the DMF solvent under the given temperature (Th). The solution gradually reaches supersaturation, and the nuclei start to form on the substrate. After the solvent is completely evaporated, the CsPbX3 perovskite single microcrystals are obtained on the substrate surface. (b) Schematic chemical form of perovskite CsPbX3 structure.
Fig. 2. Geometry of the CsPbClxBr3−x single microcrystals on different substrates. SEM micrograph of CsPbBr3 on (a) single-crystal Si, (b) sapphire, and (c) amorphous quartz, with the reactant concentration of 40 mmol/L at an evaporation temperature of 40°C. Scale bars: 50 μm. (d) EDS spectra of the CsPbCl3 and CsPbBr3 microplates. Inset: atomic ratios of Cs/Pb/Cl and Cs/Pb/Br (∼1:1:3). (e), (f) EDS elemental mapping of the corresponding CsPbCl3 and CsPbBr3 microplates, respectively.
Fig. 3. Tunable band-gap energies and emission spectra of the CsPbX3 microcrystals. (a) Tunable emission wavelength and absorption of the CsPbX3 (X=Cl, Cl/Br, Br, Br/I, and I) mixed halide systems. (b) Schematic of the frequency downconversion (1PA) PL process. (c) Molecular structure and approximate cavity dimensions of β-CD. (d) Schematic diagram to show the mechanism for the water-resistant property of the CsPbBrxI3−x (0≤x<3) with β-CD. (e) XRD patterns of the as-grown CsPbX3 mixed halide perovskites. (f) Band gaps of the CsPbX3 with composition x. The filled circles are the experimental data, and the solid lines are the linearly fitted curves.
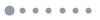
Fig. 4. Frequency downconversion single-mode lasing from CsPbClxBr3−x microcavities based on 1PA. Single-mode lasing of the CsPbBr3 (a) microplate, (b) microrod, and (c) microcube. Insets: corresponding fluorescence microscopy images of the CsPbBr3 microcrystals. (d) Integrated emission intensity and FWHM as a function of pump fluence showing the lasing threshold at ∼24.1 μJ/cm2 and gain saturation at ∼35.2 μJ/cm2. (e) Gaussian fitting of the lasing mode near the threshold, giving the FWHM of the lasing peak (δλ) ∼0.27 nm and the Q factor ∼2012. (f) Polarization characteristics of the laser emission at a pump fluence of 1.3Pth. The DOP is estimated to be 73%, corresponding to an orthogonal polarization suppression ratio of 16 dB. (g) Multicolor single-mode laser emissions and corresponding fluorescence microscopy images of the CsPbClxBr3−x microcrystals. (h) Integrated emission intensity of a CsPbBr3 microplate under 400 nm fs laser excitation at a constant pump density of 1.5Pth for over 12 h while exposed to ambient atmosphere.
Fig. 5. Nonlinear frequency upconversion single-mode lasing from CsPbX3 microcavities based on MPA. (a) Variation of the emission spectra for the CsPbBr3 microplate pumped by 800 nm (2PA). (b) Integrated emission intensity and FWHM versus pump fluence, and (c) Gaussian fitting of the lasing mode at 1.1Pth based on 2PA. (d)–(f) Single-mode lasing from CsPbBr3 microplate pumped by 1300 nm (3PA), 1800 nm (4PA), and 2300 nm (5PA), respectively. Insets: corresponding MPA and fluorescence mechanisms for the respective pump wavelengths. (g)–(j) Fluorescence microscopy images of the CsPbBr3 microrod via 5PA with increasing pump fluence, showing the transition from original SPE to lasing. (k) Pump-wavelength-dependent lasing threshold of the CsPbBr3 microrod.
Fig. 6. Lasing dynamics of the CsPbX3 microcavity lasers. (a) Streak-camera images of the CsPbBr3 microplate at the pump densities of 0.8Pth (top panel) and 1.6Pth (bottom panel). (b) Typical PL decay curve obtained at three different excitation densities of 0.5Pth, 0.9Pth, and 1.2Pth. (c) Streak-camera images and the corresponding spectral integrated waveforms of output pulses of gain-switched CsPbBr3 microcavity lasers for various pump fluences. (d) Wavelength dependence of the delay time and pulse width of the laser outputs extracted from the streak-camera image (inset) with a pump density of 1.4Pth. (e) Spectral evolution of the lasing pulses from the CsPbBr3 microplate, showing the obvious peak blueshift and linewidth broadening with increasing pump fluences from 1.05Pth to 1.95Pth. (f) Peak position and FWHM as a function of pump fluence extracted from (e).