Chao Xiang, Warren Jin, John E. Bowers, "Silicon nitride passive and active photonic integrated circuits: trends and prospects," Photonics Res. 10, A82 (2022)

Search by keywords or author
- Photonics Research
- Vol. 10, Issue 6, A82 (2022)
![Progress of silicon nitride-based photonic integration with key demonstrations labeled with the release year. The included demonstrations include SiN waveguides [27], TriPleX waveguides [29], frequency comb generation [30], ULL waveguides (<0.1 dB/m) [6], Si-SiN integration [31], ultra-high-Q ring resonators (Q>80 million) [32], hybrid integrated lasers [10], soliton generation [33], waveguides using the photonic Damascene process [34], electrically pumped comb generation [14], heterogeneously integrated lasers [12], Hz-level linewidth lasers [18], and heterogeneous laser soliton [17].](/richHtml/prj/2022/10/6/06000A82/img_001.jpg)
Fig. 1. Progress of silicon nitride-based photonic integration with key demonstrations labeled with the release year. The included demonstrations include SiN waveguides [27], TriPleX waveguides [29], frequency comb generation [30], ULL waveguides (< 0.1 dB / m ) [6], Si-SiN integration [31], ultra-high-Q ring resonators (Q > 80 million) [32], hybrid integrated lasers [10], soliton generation [33], waveguides using the photonic Damascene process [34], electrically pumped comb generation [14], heterogeneously integrated lasers [12], Hz-level linewidth lasers [18], and heterogeneous laser soliton [17].
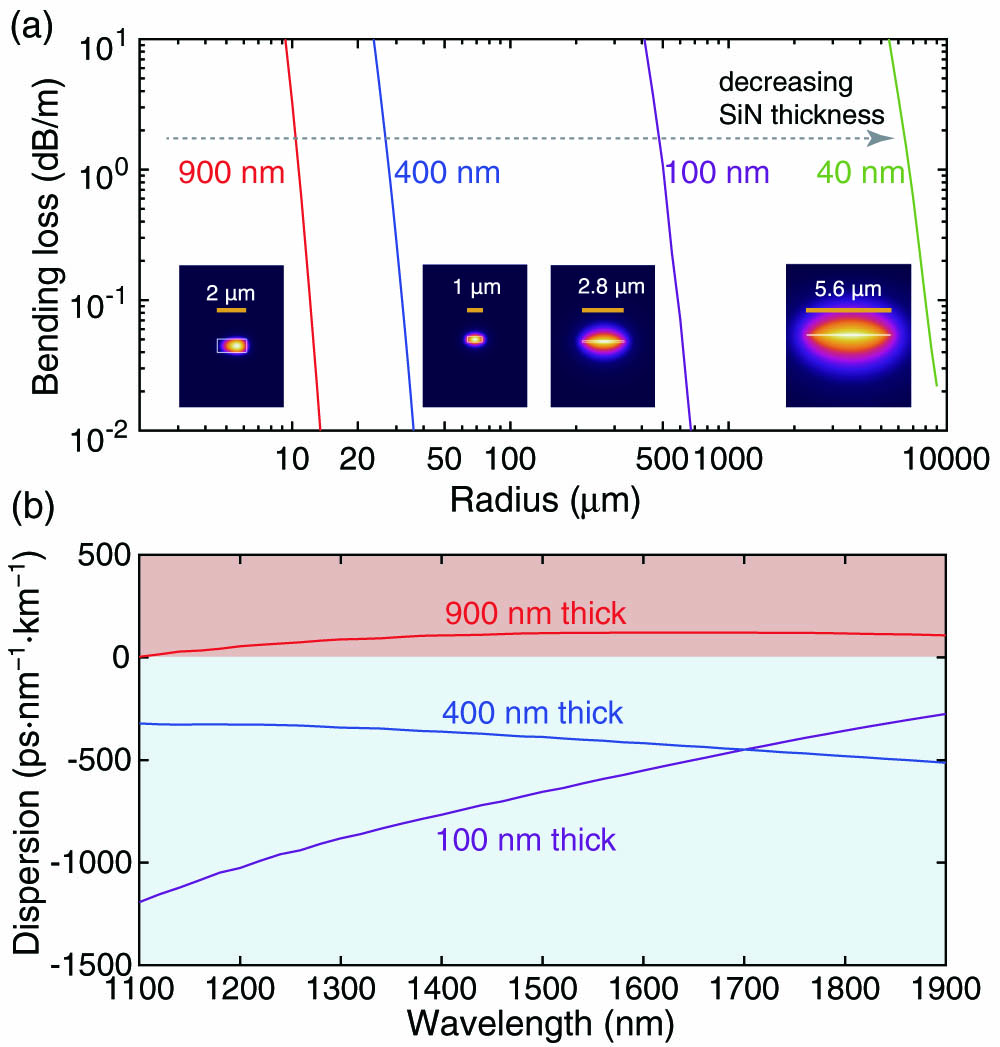
Fig. 2. (a) SiN waveguide bending loss versus bending radius of the TE0 optical mode for four different SiN core thicknesses. Insets show the mode profiles for each waveguide geometry with negligible bending loss at 20 μm, 50 μm, 1 mm, and 10 mm respectively. (b) Waveguide dispersion for waveguides with SiN core thickness and width selected from (a).
![Selected SiN-based device schematics, pictures, and results. The left column shows active devices, and the right column shows passive devices. (a) Schematics and picture of ULL SiN waveguides, 200-mm-diameter wafers, and resonators with 5 GHz and 30 GHz FSR. (b) The measured Q factors versus wavelength for the resonators shown in (a) [18]. (c) Schematics of a heterogeneous multilayer III-V/Si/SiN laser cross section [69]. (d) Device picture of heterogeneous laser soliton microcomb devices on a wafer and the optical spectrum of the generated single soliton state with 100-GHz repetition rate [17]. (e) Device picture of spiral-shaped ultra-high-Q SiN resonators with 135 MHz FSR and the measured frequency noise spectrum comparison of the self-injection locked hybrid-integrated InP/SiN laser and a fiber laser [19].](/Images/icon/loading.gif)
Fig. 3. Selected SiN-based device schematics, pictures, and results. The left column shows active devices, and the right column shows passive devices. (a) Schematics and picture of ULL SiN waveguides, 200-mm-diameter wafers, and resonators with 5 GHz and 30 GHz FSR. (b) The measured Q factors versus wavelength for the resonators shown in (a) [18]. (c) Schematics of a heterogeneous multilayer III-V/Si/SiN laser cross section [69]. (d) Device picture of heterogeneous laser soliton microcomb devices on a wafer and the optical spectrum of the generated single soliton state with 100-GHz repetition rate [17]. (e) Device picture of spiral-shaped ultra-high-Q SiN resonators with 135 MHz FSR and the measured frequency noise spectrum comparison of the self-injection locked hybrid-integrated InP/SiN laser and a fiber laser [19].
![Pictures of a few SiN-based photonic integrated circuits and devices: (a) neural probe [109], (b) multi-color engines [110], (c) biosensor with sensing window, integrated light source, and electrical interfaces [111], (d) IMEC BIOPIX 300 passive SiN chip [112], (e) SiN soliton microcomb chip [113], (f) III-V/Si/SiN laser chip [69], (g) SiN AWG and delay line chip [24], (h) hybrid-integrated turnkey soliton module [16], (i) hybrid-integrated photonic microwave beamformer module [114], and (j) quantum photonic processor chip [115].](/Images/icon/loading.gif)
Fig. 4. Pictures of a few SiN-based photonic integrated circuits and devices: (a) neural probe [109], (b) multi-color engines [110], (c) biosensor with sensing window, integrated light source, and electrical interfaces [111], (d) IMEC BIOPIX 300 passive SiN chip [112], (e) SiN soliton microcomb chip [113], (f) III-V/Si/SiN laser chip [69], (g) SiN AWG and delay line chip [24], (h) hybrid-integrated turnkey soliton module [16], (i) hybrid-integrated photonic microwave beamformer module [114], and (j) quantum photonic processor chip [115].
![(a) A comparison of the linewidth and size/cost of different types of lasers. (b) Schematics of resonator configurations for external-cavity based tunable lasers and self-injection locked-based configurations. (c) The calculated laser fundamental linewidth dependence of external-cavity lasers and self-injection locked lasers on the SiN waveguide loss. External-cavity lasers are based on Vernier SiN ring resonators with 600 μm and 626 μm radius with varying ring power coupling coefficient κ2 values. The self-injection-locked laser linewidth calculation assumes a similar configuration as in Ref. [18] with varying DFB laser to SiN high-Q resonator coupling loss. For the self-injection locking case, the SiN resonators are with coupler designs leading to loaded Q-factors approaching the intrinsic Q-factors (κ2<0.01).](/Images/icon/loading.gif)
Fig. 5. (a) A comparison of the linewidth and size/cost of different types of lasers. (b) Schematics of resonator configurations for external-cavity based tunable lasers and self-injection locked-based configurations. (c) The calculated laser fundamental linewidth dependence of external-cavity lasers and self-injection locked lasers on the SiN waveguide loss. External-cavity lasers are based on Vernier SiN ring resonators with 600 μm and 626 μm radius with varying ring power coupling coefficient κ 2 values. The self-injection-locked laser linewidth calculation assumes a similar configuration as in Ref. [18] with varying DFB laser to SiN high-Q resonator coupling loss. For the self-injection locking case, the SiN resonators are with coupler designs leading to loaded Q -factors approaching the intrinsic Q -factors (κ 2 < 0.01 ).
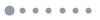
Fig. 6. Vision of multi-functional SiN-based PICs with integrated III-V gain and Si circuits.
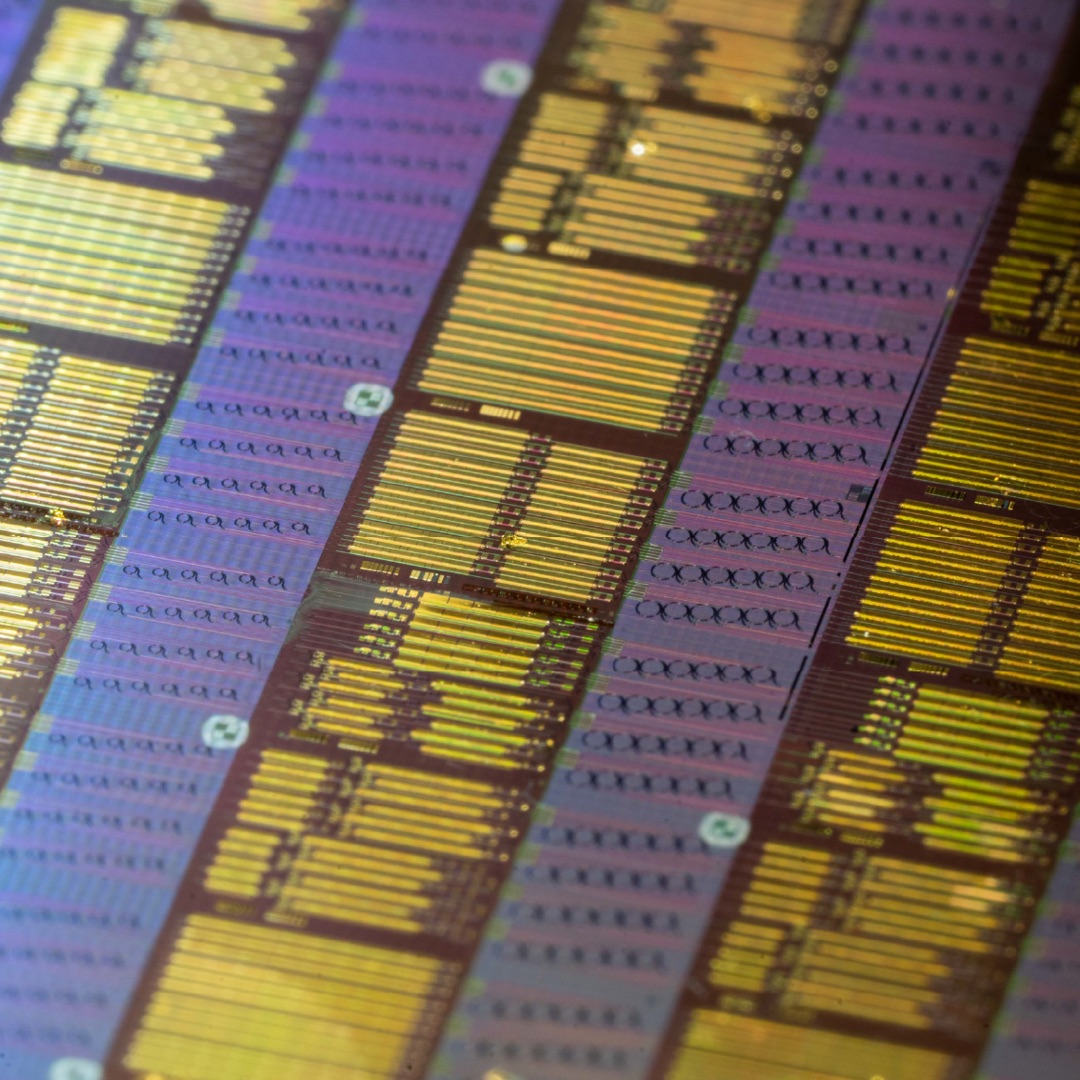
Set citation alerts for the article
Please enter your email address