
- Opto-Electronic Advances
- Vol. 6, Issue 7, 230007 (2023)
Abstract
Keywords
Introduction
Organic solid-state lasers (OSSLs) with light weight, tunable emission wavelengths, mechanical flexibility and simple fabrication processes have been intensively investigated in terms of material development, device design and mechanism study
In recent years, various strategies have been developed to construct four-level systems, such as the introduction of excimers
Considering the deficiencies in NIR organic gain materials, a general molecular design strategy of “merging two in one” was developed in this work. By merging two molecules of previously reported 2’-hydroxychalcone derivatives into one new molecule in a centrosymmetric way, a series of NIR organic gain materials were designed and synthesized, which not only dramatically expanded the conjugated systems but also provided molecular structures for the ESDPT process
Results and discussion
Molecular design and synthesis
As shown in
Figure 1.(
Photophysical properties
The ultraviolet‒visible (UV‒vis) absorption and steady state photoluminescent (PL) spectra in dichloromethane (DCM, 10−5 M) of all materials were measured at room temperature (
Fig. S2 and
To better understand the effect of our molecular strategy on the photophysical properties, the photoluminescence quantum yields (PLQYs) of these 6 molecules in DCM solutions and in PS films were carefully tested. In general, the PLQYs in PS films are higher than those in DCM solution owing to the effective suppression of molecular vibrations in PS films. The results also showed that the PLQYs of the target compounds were much higher than those of the template compounds in both DCM solutions and PS films, possibly because the stimulated emission cross-sections of the target compounds were dramatically enhanced. DHN-DJP showed the highest PLQYs (3.2% in DCM solution and 7.1% in PS film), which were much higher than those of DHN-DMP and DHN-DPP. This indicates that the julolidine group of DHN-DJP can effectively immobilize the amino group and inhibit molecular vibration
ESIPT processes
Subsequently, we studied the ESIPT processes of our target compounds. Every molecule contains two groups of resonance-assisted hydrogen bonds (RAHBs)
Figure 2.(
Next, the UV‒vis absorption and PL spectra of DMN-DMP, DMN-DPP and DMN-DJP both in DCM solutions and PS films were tested as comparation, for which no ESIPT process can occur because of the absence of RAHBs. As shown in
Figs. S8 and
Figure 3.(
To further confirm the ESDPT process, the transient absorption of DHN-DPP in PS film was measured. As shown in
Fig. S9, a small peak of the excited-state absorption (ESA) can be observed at 0.2 ps, and the intensity of the peak raised gradually before 0.6 ps. It indicates that a new excited state is formed after 0.2 ps. Interestingly, a new peak emerged after 0.4 ps, which became the major peak gradually. The intensity of the ESA peaks reached a maximum at 0.7 ps and only one broad peak can be observed because of the overlap of these two peaks. Afterwards, the intensity of the broad peak decreased gradually without new peak formed. According to the previously reported work, the ESIPT process should be very fast (kept > 10 12 s−1)
From the results of DFT calculations and experimental results, an ESDPT process of target compounds can be supposed, as shown in
Fig. S10. First, the target molecules can be excited into N* by excitation light. N* is unstable and can be converted into TA* through the first ESIPT process. TA* can go through the second reversible ESIPT process; as a result, a balance can be formed between TA* and TB*. Then, TA* and TB* fluoresce through radiative transition, generating TA and TB, respectively. TB can undergo a reverse proton transfer process to form TA, and TA can undergo a second reverse proton transfer process to form N. At this point, the ESDPT process is completed. In this process, light absorption occurs in the N form, and light emission occurs in the TA and TB forms. For TA* emissions, a four-level system can be formed, which is essential for stimulated emissions. For TB* emission, a more effective six-level system can be formed, which was proven to achieve lasing more effectively
Laser performance
To study the laser performances of our newly developed molecules, DHN-doped PS microspheres were prepared according to a previously reported method
Figure 4.(
The laser performances of DHNs were next studied with a 532 nm nanosecond pulsed laser as the pumping source. With the increase of the pumping density, a set of laser peaks with a center wavelength around 720 nm gradually emerged (
Figure 5.PL spectra of (
To investigate the laser stability of the PS microspheres doped with our new gain materials, the laser emissions were measured for 12000 pulses consecutively at a pumping density of 47.8 µJ/cm2. As shown in
Conclusions
In summary, we have developed an effective molecular design strategy for NIR organic gain materials by merging two in one, by which three new molecules, DHN-DMP, DHN-DPP and DHN-DJP, were designed and synthesized based on previously reported 2’-hydroxychalcone derivatives. All these new materials showed dramatically redshifted emission compared with their templated molecules. DFT calculations and spectral studies indicated that the redshifts are attributed to not only the expansion of conjugated systems but also the ESDPT process. We demonstrated that the first ESIPT process can proceed without any energy barrier; however, the second ESIPT process is inversible and, as a result, reaches a balance between TA* and TB*. Laser performance studies showed that all the materials can achieve NIR lasing from the TB* state, indicating the advantage of the six-level system in achieving stimulated emission. Furthermore, the structure and property relationships of our development gain materials are also systematically investigated, demonstrating that both intramolecular and intermolecular interactions can affect the laser performance. It is worth mentioning that our developed organic lasers showed high stability; in particular, the DHN-DPP-based laser can maintain high laser intensity after 12000 pulses. This study not only provided a practical method for the development of new organic laser-active materials but also demonstrated an ESDPT mechanism for NIR lasing, which will facilitate the development and practical applications of NIR organic lasers.
References
[1] Y Jiang, YY Liu, X Liu, H Lin, K Gao et al. Organic solid-state lasers: a materials view and future development. Chem Soc Rev, 49, 5885-5944(2020).
[2] AJC Kuehne, MC Gather. Organic lasers: recent developments on materials, device geometries, and fabrication techniques. Chem Rev, 116, 12823-12864(2016).
[3] CC Yan, XD Wang, LS Liao. Thermally activated delayed fluorescent gain materials: harvesting triplet excitons for lasing. Adv Sci, 9, 2200525(2022).
[4] GQ Wei, XD Wang, LS Liao. Recent advances in 1D organic solid-state lasers. Adv Funct Mater, 29, 1902981(2019).
[5] JJ Wu, XD Wang, LS Liao. Advances in energy-level systems of organic lasers. Laser Photonics Rev, 16, 2200366(2022).
[6] Y Wang, JY Yu, YF Mao, J Chen, S Wang et al. Stable, high-performance sodium-based plasmonic devices in the near infrared. Nature, 581, 401-405(2020).
[7] RM Ma, RF Oulton. Applications of nanolasers. Nat Nanotechnol, 14, 12-22(2019).
[8] GS Hong, AL Antaris, HJ Dai. Near-infrared fluorophores for biomedical imaging. Nat Biomed Eng, 1, 0010(2017).
[9] MT Hill, MC Gather. Advances in small lasers. Nat Photonics, 8, 908-918(2014).
[10] RX Yan, D Gargas, PD Yang. Nanowire photonics. Nat Photonics, 3, 569-576(2009).
[11] YC Wei, SF Wang, Y Hu, LS Liao, DG Chen et al. Overcoming the energy gap law in near-infrared OLEDs by exciton–vibration decoupling. Nat Photonics, 14, 570-577(2020).
[12] JV Caspar, EM Kober, BP Sullivan, TJ Meyer. Application of the energy gap law to the decay of charge-transfer excited states. J Am Chem Soc, 104, 630-632(1982).
[13] JJ Wu, XD Wang, LS Liao. Near-infrared solid-state lasers based on small organic molecules. ACS Photonics, 6, 2590-2599(2019).
[14] J Gierschner, S Varghese, SY Park. Organic single crystal lasers: a materials view. Adv Opt Mater, 4, 348-364(2016).
[15] C Wei, MM Gao, FQ Hu, JN Yao, YS Zhao. Excimer emission in self-assembled organic spherical microstructures: an effective approach to wavelength switchable microlasers. Adv Opt Mater, 4, 1009-1014(2016).
[16] HY Dong, YH Wei, W Zhang, C Wei, CH Zhang et al. Broadband tunable microlasers based on controlled intramolecular charge-transfer process in organic supramolecular microcrystals. J Am Chem Soc, 138, 1118-1121(2016).
[17] YH Wei, HY Dong, C Wei, W Zhang, YL Yan et al. Wavelength-tunable microlasers based on the encapsulation of organic dye in metal–organic frameworks. Adv Mater, 28, 7424-7429(2016).
[18] K Wang, ZH Gao, W Zhang, YL Yan, HW Song et al. Exciton funneling in light-harvesting organic semiconductor microcrystals for wavelength-tunable lasers. Sci Adv, 5, eaaw2953(2019).
[19] D Okada, S Azzini, H Nishioka, A Ichimura, H Tsuji et al. π-Electronic co-crystal microcavities with selective vibronic-mode light amplification: toward förster resonance energy transfer lasing. Nano Lett, 18, 4396-4402(2018).
[20] HT Lin, YX Ma, S Chen, XD Wang. Hierarchical integration of organic core/shell microwires for advanced photonics. Angew Chem Int Ed, 62, e202214214(2023).
[21] Q Lv, XD Wang, Y Yu, MP Zhuo, M Zheng et al. Lattice-mismatch-free growth of organic heterostructure nanowires from cocrystals to alloys. Nat Commun, 13, 3099(2022).
[22] YX Ma, S Chen, HT Lin, SP Zhuo, XD Wang. Organic low-dimensional crystals undergoing twinning deformation. Sci Bull, 67, 1632-1635(2022).
[23] Y Su, ZF Yao, B Wu, YD Zhao, JY Han et al. Organic polymorph-based alloys for continuous regulation of emission colors. Matter, 5, 1520-1531(2022).
[24] W Zhang, YL Yan, JM Gu, JN Yao, YS Zhao. Low-threshold wavelength-switchable organic nanowire lasers based on excited-state intramolecular proton transfer. Angew Chem Int Ed, 54, 7125-7129(2015).
[25] X Cheng, K Wang, S Huang, HY Zhang, HY Zhang et al. Organic crystals with near-infrared amplified spontaneous emissions based on 2’-hydroxychalcone derivatives: subtle structure modification but great property change. Angew Chem Int Ed, 54, 8369-8373(2015).
[26] CC Yan, XD Wang, LS Liao. Organic lasers harnessing excited state intramolecular proton transfer process. ACS Photonics, 7, 1355-1366(2020).
[27] VS Padalkar, S Seki. Excited-state intramolecular proton-transfer (ESIPT)-inspired solid state emitters. Chem Soc Rev, 45, 169-202(2016).
[28] JE Kwon, SY Park. Advanced organic optoelectronic materials: harnessing excited-state intramolecular proton transfer (ESIPT) process. Adv Mater, 23, 3615-3642(2011).
[29] P Chou, D McMorrow, TJ Aartsma, M Kasha. The proton-transfer laser. Gain spectrum and amplification of spontaneous emission of 3-hydroxyflavone. J Phys Chem, 88, 4596-4599(1984).
[30] GQ Wei, Y Yu, MP Zhuo, XD Wang, LS Liao. Organic single-crystalline whispering-gallery mode microlasers with efficient optical gain activated via excited state intramolecular proton transfer luminogens. J Mater Chem C, 8, 11916-11921(2020).
[31] WY Yang, RC Lai, JJ Wu, YJ Yu, CC Yan et al. Deepening insights into near-infrared excited-state intramolecular proton transfer lasing: the charm of resonance-assisted hydrogen bonds. Adv Funct Mater, 32, 2204129(2022).
[32] VTN Mai, A Shukla, M Mamada, S Maedera, PE Shaw et al. Low amplified spontaneous emission threshold and efficient electroluminescence from a carbazole derivatized excited-state intramolecular proton transfer dye. ACS Photonics, 5, 4447-4455(2018).
[33] KY Chen, CC Hsieh, YM Cheng, CH Lai, PT Chou. Extensive spectral tuning of the proton transfer emission from 550 to 675 nm via a rational derivatization of 10-hydroxybenzo[h]quinoline. Chem Commun, 42, 4395-4397(2006).
[34] XD Wang, Q Liao, XM Lu, H Li, ZZ Xu et al. Shape-engineering of self-assembled organic single microcrystal as optical microresonator for laser applications. Sci Rep, 4, 7011(2014).
[35] X Cheng, YF Zhang, SH Han, F Li, HY Zhang et al. Multicolor amplified spontaneous emissions based on organic polymorphs that undergo excited-state intramolecular proton transfer. Chem Eur J, 22, 4899-4903(2016).
[36] ZL Che, CC Yan, XD Wang, LS Liao. Organic near-infrared luminescent materials based on excited state intramolecular proton transfer process. Chin J Chem, 40, 2468-2481(2022).
[37] XD Wang, Q Liao, H Li, SM Bai, YS Wu et al. Near-infrared lasing from small-molecule organic hemispheres. J Am Chem Soc, 137, 9289-9295(2015).
[38] XD Wang, ZZ Li, MP Zhuo, YS Wu, S Chen et al. Tunable near-infrared organic nanowire nanolasers. Adv Funct Mater, 27, 1703470(2017).
[39] XD Wang, ZZ Li, SF Li, H Li, JW Chen et al. Near-infrared organic single-crystal lasers with polymorphism-dependent excited state intramolecular proton transfer. Adv Opt Mater, 5, 1700027(2017).
[40] JJ Wu, HF Gao, RC Lai, MP Zhuo, JG Feng et al. Near-infrared organic single-crystal nanolaser arrays activated by excited-state intramolecular proton transfer. Matter, 2, 1233-1243(2020).
[41] D Venkatakrishnarao, YSLV Narayana, MA Mohaiddon, EA Mamonov, N Mitetelo et al. Two-photon luminescence and second-harmonic generation in organic nonlinear surface comprised of self-assembled frustum shaped organic microlasers. Adv Mater, 29, 1605260(2017).
[42] JJ Wu, MP Zhuo, RC Lai, SN Zou, CC Yan et al. Cascaded excited-state intramolecular proton transfer towards near-infrared organic lasers beyond 850 nm. Angew Chem Int Ed, 60, 9114-9119(2021).
[43] CC Yan, YP Liu, WY Yang, JJ Wu, XD Wang et al. Excited-state intramolecular proton transfer parent core engineering for six-level system lasing toward 900 nm. Angew Chem Int Ed, 61, e202210422(2022).
[44] WY Yang, CC Yan, XD Wang, LS Liao. Recent progress on the excited-state multiple proton transfer process in organic molecules. Sci China Chem, 65, 1843-1853(2022).
[45] R Aoki, R Komatsu, K Goushi, M Mamada, SY Ko et al. Realizing near-infrared laser dyes through a shift in excited-state absorption. Adv Opt Mater, 9, 2001947(2021).
[46] CC Yan, JJ Wu, WY Yang, S Chen, Q Lv et al. Precise synthesis of multilevel branched organic microwires for optical signal processing in the near infrared region. Sci China Mater, 65, 1020-1027(2022).
[47] WY Mao, J Tang, LQ Dai, XY He, J Li et al. A general strategy to design highly fluorogenic far-red and near-infrared tetrazine bioorthogonal probes. Angew Chem Int Ed, 60, 2393-2397(2021).
[48] SJ Lim, J Seo, SY Park. Photochromic switching of excited-state intramolecular proton-transfer (ESIPT) fluorescence: a unique route to high-contrast memory switching and nondestructive readout. J Am Chem Soc, 128, 14542-14547(2006).
[49] ZY Zhang, YA Chen, WY Hung, WF Tang, YH Hsu et al. Control of the reversibility of excited-state intramolecular proton transfer (ESIPT) reaction: host-polarity tuning white organic light emitting diode on a new thiazolo[5, 4-d]thiazole ESIPT system. Chem Mater, 28, 8815-8824(2016).
[50] TEA Frizon, CAM Salla, F Grillo, FS Rodembusch, VS Câmara et al. ESIPT-based benzazole-pyromellitic diimide derivatives. A thermal, electrochemical, and photochemical investigation. Spectrochim Acta A Mol Biomol Spectrosc, 288, 122050(2023).
[51] CY Peng, JY Shen, YT Chen, PJ Wu, WY Hung et al. Optically triggered stepwise double-proton transfer in an intramolecular proton relay: a case study of 1, 8-dihydroxy-2-naphthaldehyde. J Am Chem Soc, 137, 14349-14357(2015).
[52] PM Vérité, CA Guido, D Jacquemin. First-principles investigation of the double ESIPT process in a thiophene-based dye. Phys Chem Chem Phys, 21, 2307-2317(2019).
[53] T Wróblewski, D Ushakou. Stepwise excited-state double proton transfer and fluorescence decay analysis. J Fluoresc, 33, 103-111(2023).
[54] GQ Wei, XD Wang, LS Liao. Recent advances in organic whispering-gallery mode lasers. Laser Photonics Rev, 14, 2000257(2020).
[55] D Venkatakrishnarao, EA Mamonov, TV Murzina, R Chandrasekar. Advanced organic and polymer whispering-gallery-mode microresonators for enhanced nonlinear optical light. Adv Optical Mater, 6, 1800343(2018).
[56] XD Wang, Q Liao, QH Kong, Y Zhang, ZZ Xu et al. Whispering-gallery-mode microlaser based on self-assembled organic single-crystalline hexagonal microdisks. Angew Chem Int Ed, 53, 5863-5867(2014).
[57] T Matsushima, S Yoshida, K Inada, Y Esaki, T Fukunaga et al. Degradation mechanism and stability improvement strategy for an organic laser gain material 4, 4'-Bis[(N-carbazole)styryl]biphenyl (BSBCz). Adv Funct Mater, 29, 1807148(2019).
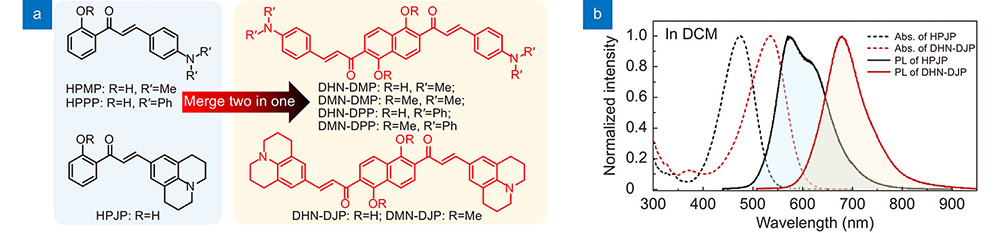
Set citation alerts for the article
Please enter your email address