Baoqi Shi, Yi-Han Luo, Wei Sun, Yue Hu, Jinbao Long, Xue Bai, Anting Wang, Junqiu Liu, "Frequency-comb-linearized, widely tunable lasers for coherent ranging," Photonics Res. 12, 663 (2024)

Search by keywords or author
- Photonics Research
- Vol. 12, Issue 4, 663 (2024)
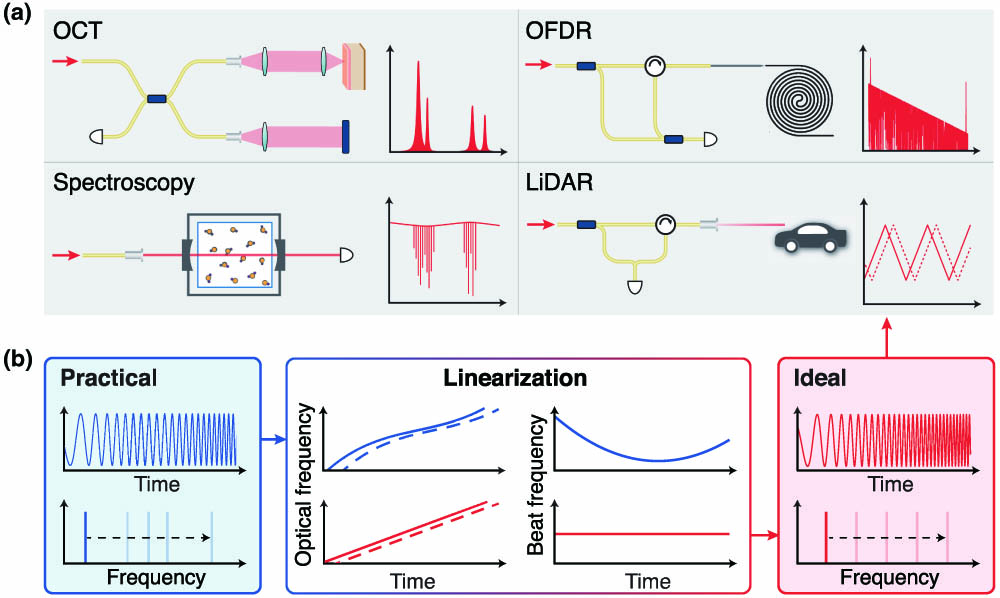
Fig. 1. Applications and principle of widely tunable lasers. (a) Applications requiring linearly chirping lasers. OCT, optical coherence tomography; OFDR, optical frequency-domain reflectometry; LiDAR, light detection and ranging. (b) Principle of laser chirp linearization. An ideal laser chirps at a constant rate. However, in reality, the actual chirp rate varies. By beating the laser with its delayed part, the chirp nonlinearity in the optical domain is revealed in the radio frequency (RF) domain.
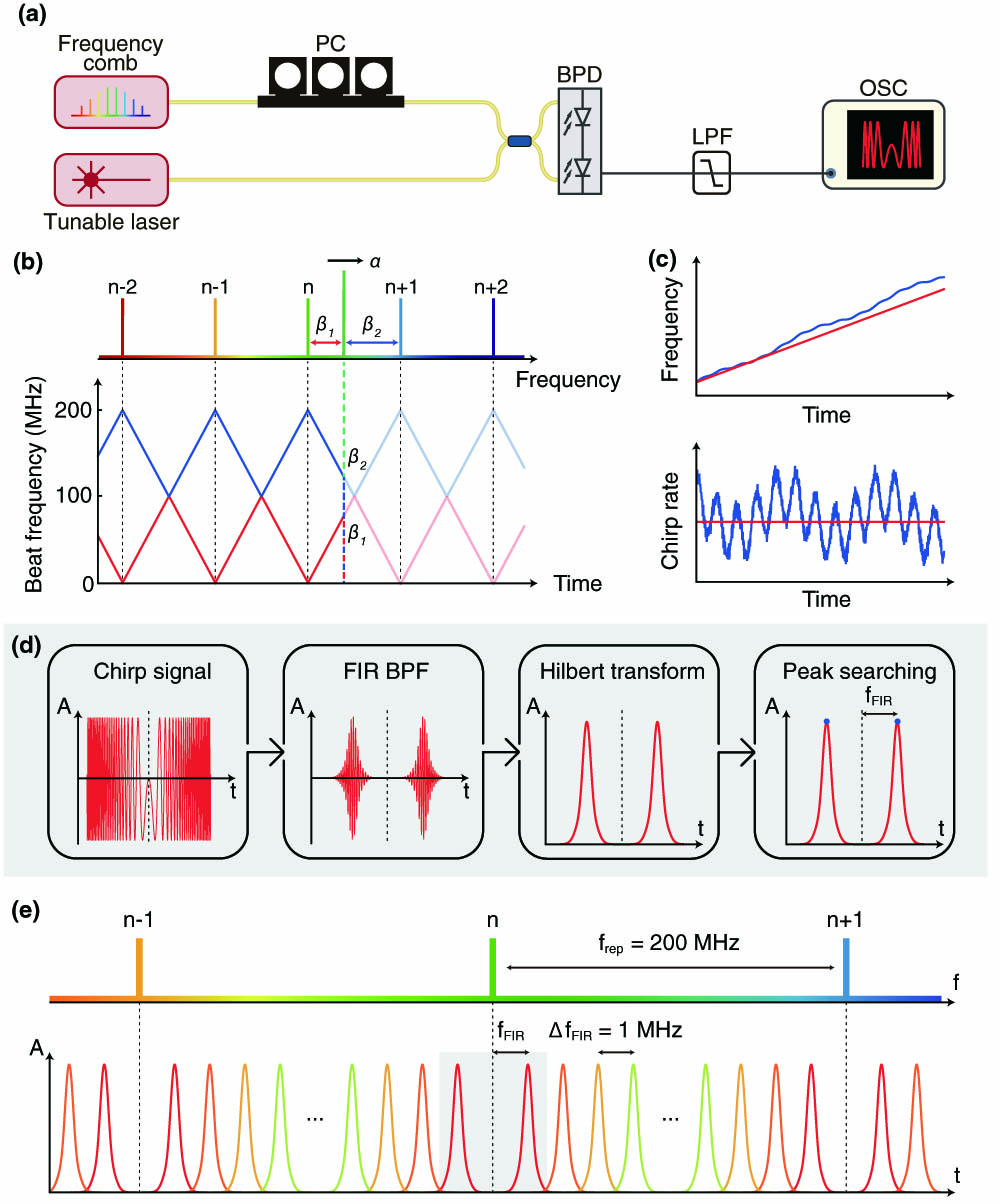
Fig. 2. Schematic and experimental setup of laser chirp characterization. (a) Experimental setup. BPF, band-pass filter; PC, polarization controller; BPD, balanced photodetector; LPF, low-pass filter; OSC, oscilloscope. (b) Illustration of the laser frequency beating with the OFC during laser chirping at a rate of α . The time traces of the two beat frequencies β 1 and β 2 (β 2 > β 1 ) are shown. (c) Upper panel shows the instantaneous frequency of the tunable laser in the ideal (red) and actual (blue) cases. Lower panel shows the corresponding instantaneous chirp rate α . (d) Flowcharts of the algorithm based on finite impulse response (FIR) band-pass filters (BPFs), to extract the instantaneous laser frequency as well as the chirp rate. The dashed lines mark the time where the laser frequency scans across a comb line. (e) The FIR filter’s center frequency f FIR is digitally set, and the instantaneous laser frequency is calculated over 1 MHz intervals.
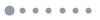
Fig. 3. Characterization of Toptica CTL laser’s chirp dynamics. (a) Normalized chirp rate α ( λ ) / α set with different set values α set over 0.2 nm wavelength range. (b) Frequency spectra of the normalized chirp rate α ( t ) / α set . The frequency values of prominent peaks are marked. (c) Normalized chirp rate α ( λ ) / α set with different set values α set over 10 nm wavelength range.
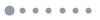
Fig. 4. Coherent LiDAR experiment. (a) Maps of measured relative distance of an engraved surface with pre-linearization and without linearization. The sample’s tilt angle of 11.6° is measured and subtracted. (b) Space spectra of the ranging profiles obtained with pre-linearization and without linearization. Fast Fourier transform is applied on the ranging profile of each case to retrieve the spectrum. The peaks correspond to the distance d = 4 m of the surface to the laser.
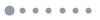
Fig. 5. Comparison of three Toptica lasers’ chirp dynamics in the wide tuning mode. (a) Normalized chirp rate α ( λ ) / α set of the three Toptica lasers with different set values α set over 0.2 nm wavelength range. (b) Frequency spectra of the chirp rate α ( t ) / α set .
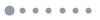
Fig. 6. Characterization of chirp dynamics of a Santec laser in the wide tuning mode. (a) Normalized chirp rate α ( λ ) / α set with different set values α set over 0.2 nm wavelength range. (b) Frequency spectra of the chirp rate α ( t ) / α set . (c) Normalized chirp rate α ( λ ) / α set with α set = 100 nm / s over 10 nm wavelength range.
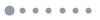
Fig. 7. Comparison of four Santec lasers’ chirp dynamics in the wide tuning mode. (a) Normalized chirp rate α ( λ ) / α set of the four Santec lasers with α set = 5 nm / s over 0.2 nm wavelength range. (b) Frequency spectra of the chirp rate α ( t ) / α set .
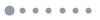
Fig. 8. Characterization of chirp dynamics of a New Focus laser in the wide tuning mode. (a) Normalized chirp rate α ( λ ) / α set with different set values α set over 0.2 nm wavelength range. (b) Frequency spectra of the chirp rate α ( t ) / α set . (c) Normalized chirp rate α ( λ ) / α set with different set values α set over 10 nm wavelength range.
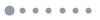
Fig. 9. Characterization of chirp dynamics of an EXFO laser in the wide tuning mode. (a) Normalized chirp rate α ( λ ) / α set with different set values α set over 0.2 nm wavelength range. (b) Frequency spectra of the chirp rate α ( t ) / α set .
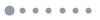
Fig. 10. Characterization of chirp dynamics of an NKT laser in the fine tuning mode. (a) Normalized chirp rate α ( f l ) / α set with different set values f mod over 6 GHz frequency range. The piezo of the laser is driven by a triangular (blue) or a sinusoidal (red) signal. (b) Frequency spectra of the chirp rate α ( t ) / α set .
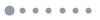
Fig. 11. Characterization of chirp dynamics of two NKT lasers in the fine tuning mode. (a) Normalized chirp rate α ( f l ) / α set with f mod = 10 Hz over 6 GHz frequency range. The piezo of the laser is driven by a triangular (blue) or a sinusoidal (red) signal. (b) Frequency spectra of the chirp rate α ( t ) / α set .
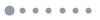
Fig. 12. Characterization of chirp dynamics of a Santec laser in the fine tuning mode. (a) Normalized chirp rate α ( f l ) / α set with different set values f mod over 9 GHz frequency range. The piezo of the laser is driven by a triangular (blue) or a sinusoidal (red) signal. (b) Frequency spectra of the chirp rate α ( t ) / α set .
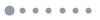
Fig. 13. Characterization of chirp dynamics of four Santec lasers in the fine tuning mode. (a) Normalized chirp rate α ( f l ) / α set with f mod = 2 Hz over 9 GHz frequency range. The piezo of the laser is driven by a triangular (blue) or a sinusoidal (red) signal. (b) Frequency spectra of the chirp rate α ( t ) / α set .
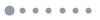
Fig. 14. Characterization of chirp dynamics of a Toptica laser in the fine tuning mode. (a) Normalized chirp rate α ( f l ) / α set with different set values f mod over 9 GHz frequency range. The piezo of the laser is driven by a triangular (blue) or a sinusoidal (red) signal. (b) Frequency spectra of the chirp rate α ( t ) / α set .
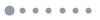
Fig. 15. (a) Different drive signals. (b) Fourier transform of different drive signals.
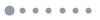
Fig. 16. Characterization of chirp dynamics of three Toptica lasers in the fine tuning mode. (a) Normalized chirp rate α ( f l ) / α set with f mod = 50 Hz over 9 GHz frequency range. The piezo of the laser is driven by a triangular (blue) or a sinusoidal (red) signal. (b) Frequency spectra of the chirp rate α ( t ) / α set .
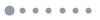
Fig. 17. Characterization of chirp dynamics of a New Focus laser in the fine tuning mode. (a) Normalized chirp rate α ( f l ) / α set with different set values f mod over 9 GHz frequency range. The piezo of the laser is driven by a triangular (blue) or a sinusoidal (red) signal. (b) Frequency spectra of the chirp rate α ( t ) / α set .
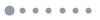
Fig. 18. Laser characterization for LiDAR demonstration. (a) Normalized chirp rate α ( f l ) / α set with different set values f mod over 24 GHz frequency range. The piezo of the laser is driven by a triangular (blue) or a sinusoidal (red) signal. (b) Frequency spectra of the chirp rate α ( t ) / α set . (c) Upper panel shows the chirp rate curve α ( t ) (blue) and pre-linearization curve α c ( t ) (red). Lower panel shows the linearized chirp rate curve α ( t ) / α c ( t ) . (d) Frequency spectrum of the linearized chirp rate in the lower panel of panel (c). The dotted and dashed line shows its averaged amplitude below 100 kHz. The dashed line shows the averaged amplitude of normalized chirp rate α ( t ) / α set ( t ) ’s frequency spectrum below 100 kHz.
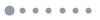
Fig. 19. Setup for LiDAR demonstration and precision test. (a) Experimental setup. BPD, balanced photodetector; OSC, oscilloscope. (b) Histogram of deviation of ranging measurement. The long-term stability of pre-linearization is verified by a precision test. A mirror is fixed at a distance of 53.361 mm and measured 1128 times every 3 s. The standard deviation of the measured distance is 11 μm.
|
Table 1. Comparison of Laser Chirp Dynamics of Different Lasers with Different Conditions
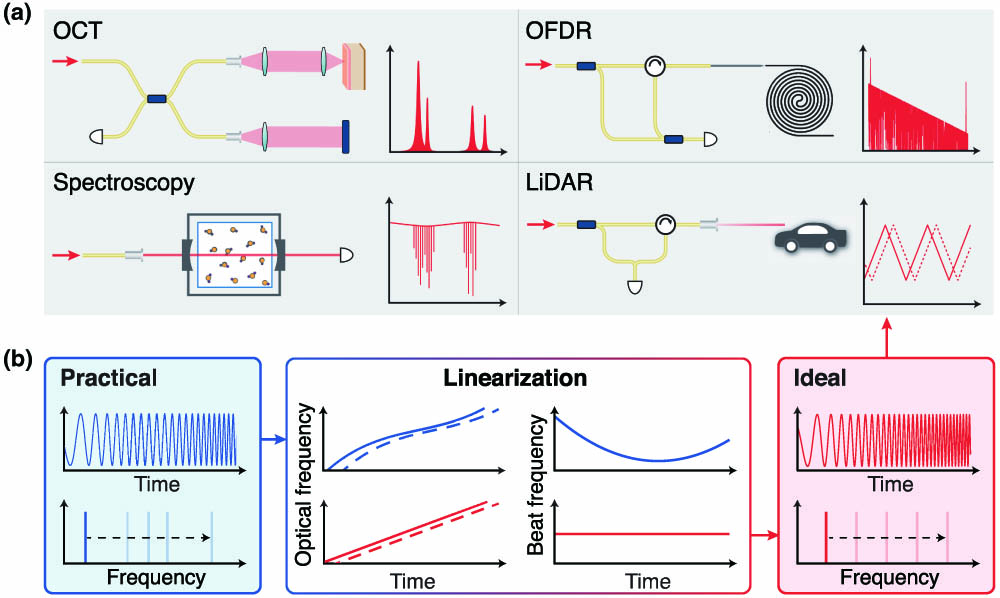
Set citation alerts for the article
Please enter your email address