Xinyuan Fang, Haocheng Yang, Wenzhe Yao, Tianxin Wang, Yong Zhang, Min Gu, Min Xiao, "High-dimensional orbital angular momentum multiplexing nonlinear holography," Adv. Photon. 3, 015001 (2021)

Search by keywords or author
- Advanced Photonics
- Vol. 3, Issue 1, 015001 (2021)
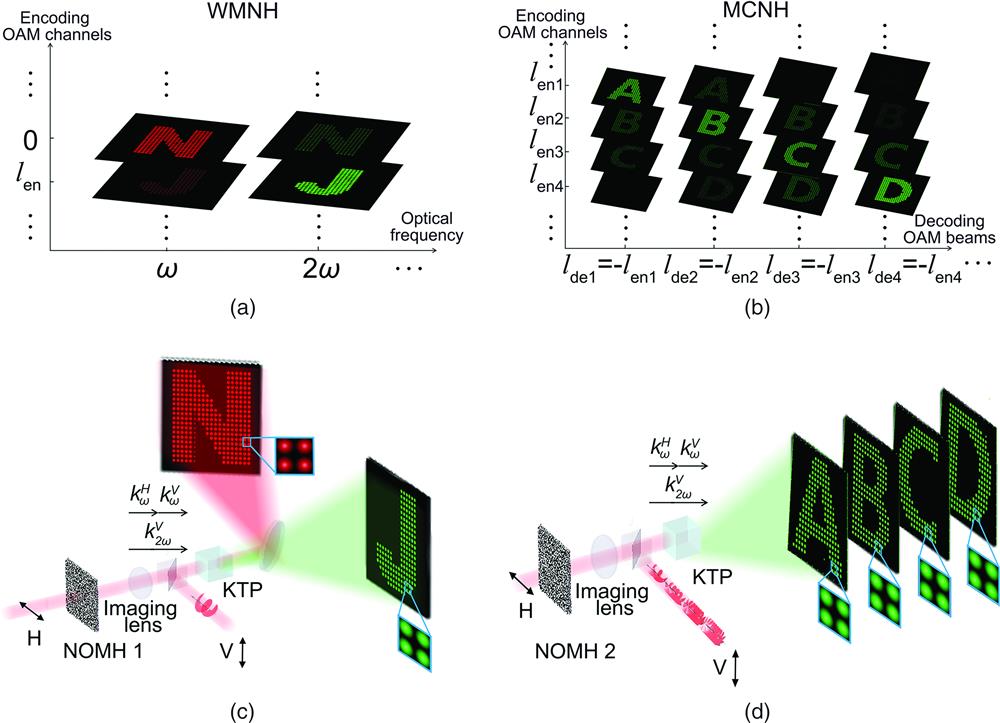
Fig. 1. Principle of OMNH and its applications in type-II SHG. (a), (b) Concepts of (a) WMNH and (b) MCNH. (c) Schematic diagram of NOMH1 capable of independently reconstructing pattern N in FW and pattern J in SHW based on WMNH in a type-II SHG process. (d) Schematic diagram of NOMH2 capable of reconstructing patterns A, B, C, and D in SHW based on MCNH in a type-II SHG process.
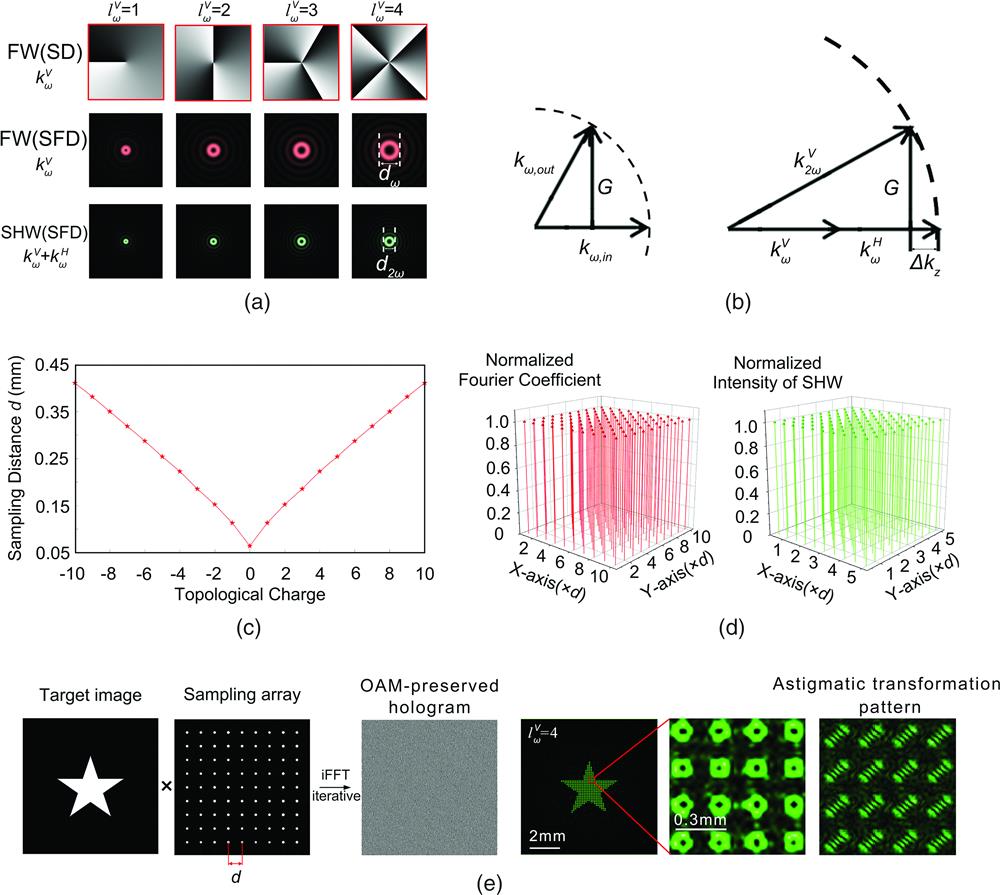
Fig. 2. Designing principle of OAM-preserved hologram in OMNH. (a) The FW sampling distance ( ) and SHW sampling distance ( ) calculated from spatial frequencies of the corresponding OAM beams in SFD. (b) The linear (left) and nonlinear (right) Raman–Nath processes in -space. Note that is significantly enlarged for clarity. The actual reciprocal vector is two-orders of magnitude less than the wave vectors in our experiment. (c) The dependence of the sampling distance for OAM-preserved hologram in OMNH on the topological charge of the FW OAM beam. (d) Taking into account , we calculate the normalized SH intensity distribution (right) reconstructed from a hologram with uniform Fourier coefficients (left). Clearly, the uniform SH intensity indicates a negligible influence from (see Note S4 in the Supplemental Materials for details). (e) Under the scheme in Fig. 1(c) , we numerically design an OAM-preserved hologram for a star (left) and calculate the reconstructed SH image (right). The FW OAM beam carries a topological charge of . The intensity profile of each pixel presents a donut shape. The corresponding astigmatic transformation pattern of each pixel well proves the OAM preservation.
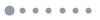
Fig. 3. Experimental demonstration of NOMH for WMNH. (a) Experimental setup for reconstructing FW and SHW images. LP, linear polarizer; SLM1/SLM2, spatial light modulator; L1-L5, lens; BS, beam splitter; PBS, polarization beam splitter; HWP, half-wave plate; DM, dichroic mirror. (b) FW (left) and SHW (right) images reconstructed by a decoding FW OAM beam with . (c) The intensity distribution of all the pixels in (b). The image pixels are numbered according to top-to-bottom and left-to-right sequences.
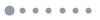
Fig. 4. Demonstrations of OAM- and polarization-dependent MCNH in SHW. (a) The images in the SHW channels are reconstructed by the vertically polarized decoding FW with , 4, 6, 8, respectively (top). (b) The intensities of all of the pixels in four channels are analyzed. The image pixels are numbered according to top-to-bottom and left-to-right sequences.
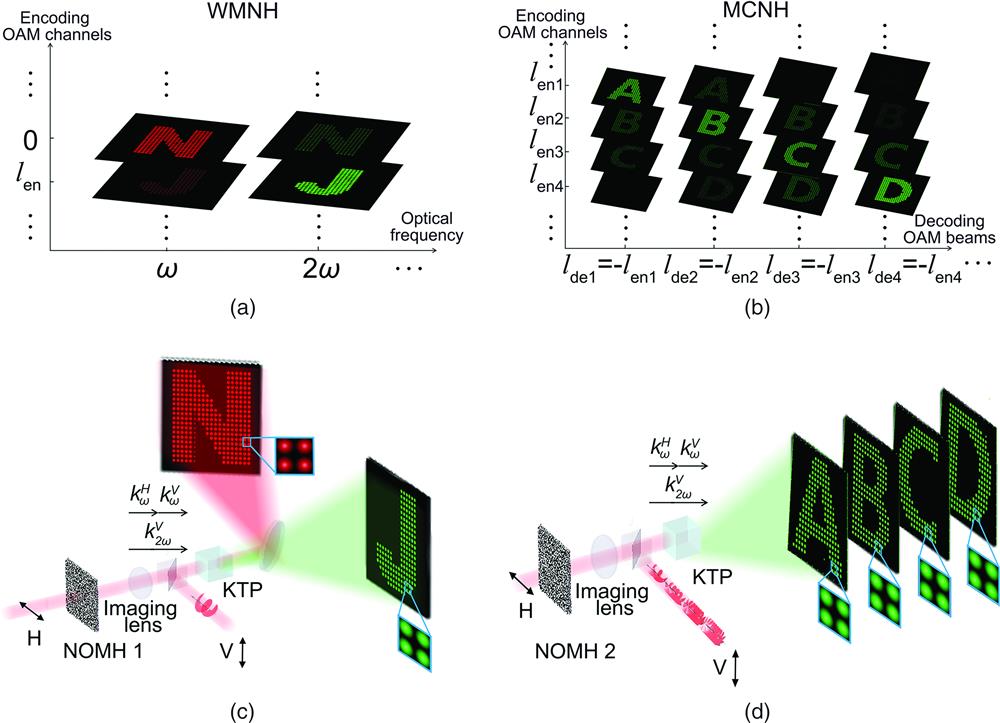
Set citation alerts for the article
Please enter your email address