Yuhang Li, Tianyi Gan, Bijie Bai, Çağatay Işıl, Mona Jarrahi, Aydogan Ozcan, "Optical information transfer through random unknown diffusers using electronic encoding and diffractive decoding," Adv. Photon. 5, 046009 (2023)

Search by keywords or author
- Advanced Photonics
- Vol. 5, Issue 4, 046009 (2023)
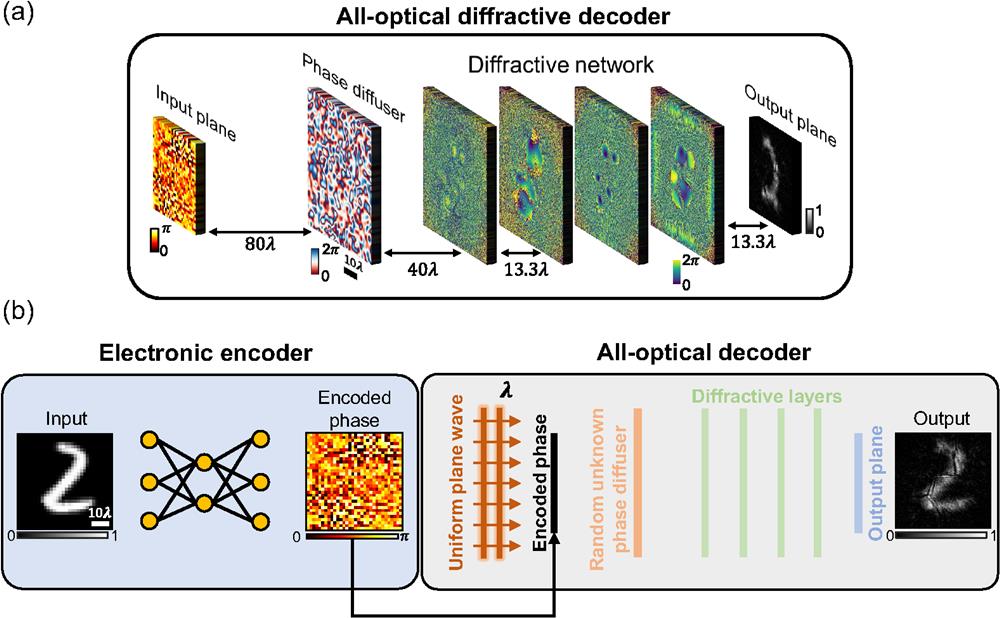
Fig. 1. Pipeline of the hybrid electronic encoder and optical diffractive decoder for optical information transfer through random unknown diffusers. (a) Schematic drawing of the presented diffractive decoder, which all-optically decodes the encoded information distorted by random phase diffusers without the need for a digital computer. (b) Workflow of the hybrid electronic-optical model: the electronic neural network encodes the input objects into 2D phase patterns and the all-optical diffractive neural network decodes the information transmitted through random, unknown phase diffusers.
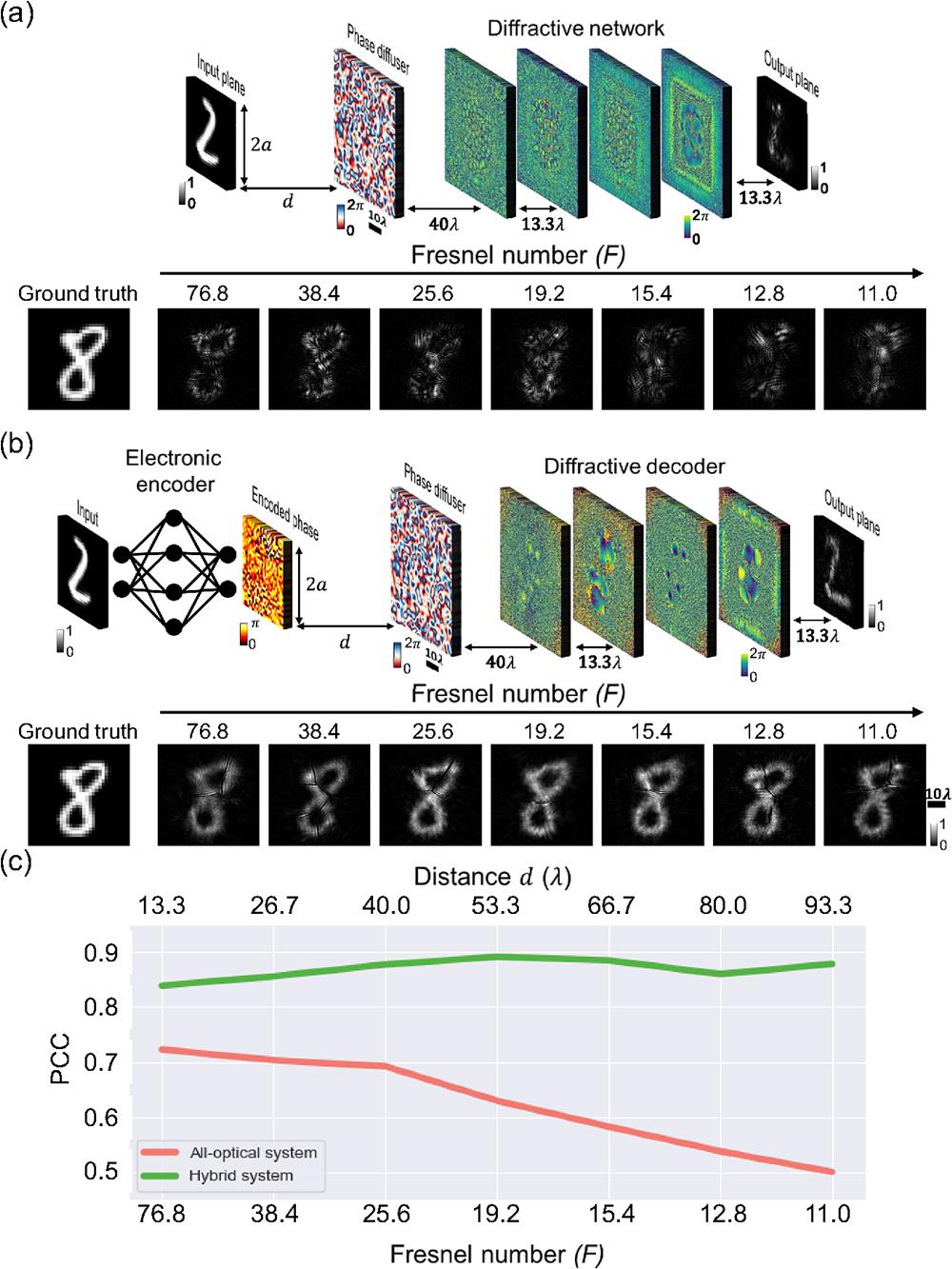
Fig. 2. Comparison between all-optical diffractive networks and the hybrid electronic-optical models for transferring optical information through random unknown diffusers. (a) Schematic of a four-layer diffractive network trained to all-optically reconstruct the amplitude images of input objects through random phase diffusers without electronic encoding. (b) Schematic of a four-layer diffractive decoder with an electronic encoder jointly trained to decode the encoded optical image through random unknown diffusers. (c) The information transmission fidelity (PCC) of the two approaches (all-optical versus hybrid) as a function of the Fresnel number ( ) of the diffractive system. All the parameters were identical in the two approaches being compared, except for the existence of the electronic encoder (at the front end of the hybrid approach).
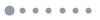
Fig. 3. Optical information transfer through random phase diffusers using electronic encoding and diffractive decoding. (a) Random phase diffusers with a correlation length used for training and testing the presented joint electronic-optical model. (b) Phase profiles of the trained diffractive layers of the optical decoder. (c) Examples of optical information encoded by an electronic-encoder CNN that is jointly trained with an optical decoder; output images processed by the all-optical decoder are also shown on the right. (d) Sample images captured by an aberration-free diffraction-limited lens.
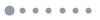
Fig. 4. Simulation results of the hybrid electronic-optical model for optical information transmission through unknown random phase diffusers. .
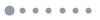
Fig. 5. Additional trainable diffractive layers (increasing ) improve the optical information transfer performance through random unknown diffusers. The PCC values are listed for each transmitted message.
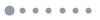
Fig. 6. The impact of the phase bit depth on the optical information transfer fidelity. (a) The joint model trained without any bit-depth limitations, i.e., 16-bit phase representation, and tested with restricted bit-depth phase patterns and decoder pairs. The average PCC value for 10,000 handwritten test digits transmitted through unknown (new) random diffusers is provided in each case. (b) The resulting mean PCC values are compared for various combinations of phase bit-depth. .
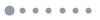
Fig. 7. The electronic encoder and the diffractive decoder trained and tested with different levels of phase bit-depth. The average PCC values are listed for each case. .
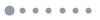
Fig. 8. Experimental demonstration of optical information transfer through an unknown random diffuser using a jointly trained pair of an electronic encoder and an optical decoder. (a) Schematic of the joint model for experimental demonstration. (b) Left: height profiles of a new random diffuser (never seen before) and the trained diffractive layers of the all-optical decoder. Right: the fabricated new random diffuser and the diffractive layers used in the experiment. (c) Schematic of the terahertz system. (d) Photograph of the experimental setup and the 3D-printed diffractive decoder.
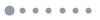
Fig. 9. Experimental results of optical information transfer through an unknown random phase diffuser using the 3D-printed diffractive decoder with electronic encoding.
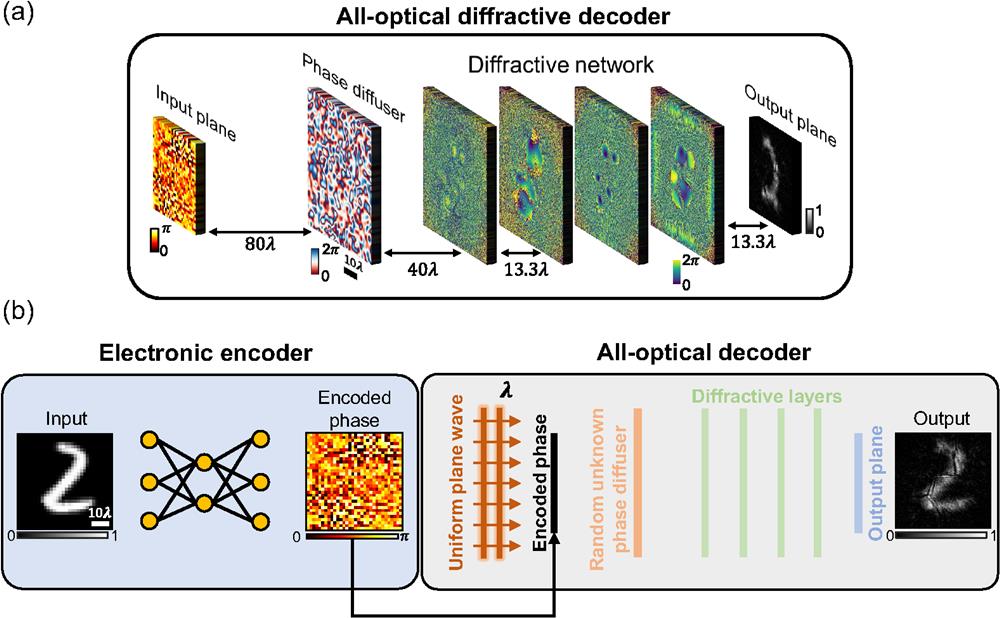
Set citation alerts for the article
Please enter your email address