
- Chinese Optics Letters
- Vol. 19, Issue 12, 121404 (2021)
Abstract
1. Introduction
Visible laser diodes (LDs) based on group III nitride materials have been employed as light sources in many fields such as full-color laser projection, laser lighting, under water communication, and material processing[
Distributed polarization doping (DPD) is a newly proposed technique that can provide holes in nitride materials without Mg doping[
In this article, we designed and fabricated GaN-based green LDs with low threshold current density by employing DPD
2. Experimental Details
Two LD structures (named Polar. and Ref. LDs, respectively) were designed, as shown in Fig. 1(a). Low growth temperature and a hybrid
Figure 1.(a) Schematic structures of green Polar. and Ref. LDs, respectively. (b) Sample structure for Hall measurements, the dash line shows the current pathway in measurement.
The theoretical hole concentration
3. Results and Discussion
As the composition-graded layer in Polar. LDs will lead to the anti-waveguide structure, which may influence the optical confinement factor (
Figure 2.Simulated optical field distribution of (a) LDs with DPD p-CL and (b) LDs with Mg doping p-CL. Insets show the enlarged distribution around p-CL.
Polar. and Ref. structures, respectively, were grown by metal organic chemical vapor deposition. Inductively coupled plasma dry etching was used to form the ridge waveguide LDs. A 200 nm Si dioxide layer was deposited as the insulating layer using inductively coupled plasma chemical vapor deposition on both sides of the ridges. A 200 nm indium tin oxide (ITO) layer was then deposited on top of the ridge using electron beam evaporation. About 100/500 nm of titanium (Ti)/Au was then deposited on top of ITO as a
Figure 3.(a) Power-current (P-I) curves and (b) laser spectra of green LDs with DPD and conventional Mg doping p-CL, respectively. The solid line in (a) is a guide for the eye.
Then, the reason for improved threshold current density and deteriorative slope efficiency was explored. According to Refs. [24,25], the threshold current density and slope efficiency of the LDs could be expressed as follows:
Applying Eq. (8), the total internal loss in Polar. and Ref. LDs was
Since we have confirmed Polar. LDs have smaller internal loss, it is reasonable to speculate that the decreasing injection efficiency is the reason for deteriorative slope efficiency according to Eq. (6). Figure 4 shows the temperature dependent photoluminescence (TDPL) result of Polar. LDs, which indicates that the internal quantum efficiency is 50%. Thus, the injection efficiency is 22%. This value is pretty low compared with the estimated one in the calculations (90%). Actually, GaN-based LDs with DPD
Figure 4.TDPL result of Polar. LD. The solid line is the result of Arrhenius fitting.
Figure 5.Current-voltage (I-V) curves of green LDs with DPD and Mg doping p-CL, respectively.
The cause for low injection efficiency has not been figured out yet, and some suppositions have been given in literatures. The consumption induced by point defects in Al-rich AlGaN had been attributed to one of the reasons in UV LDs[
4. Conclusions
GaN-based green LDs with DPD and Mg doping
References
[1] H. Wang, Y. Kawahito, R. Yoshida, Y. Nakashima, K. Shiokawa. Development of a high-power blue laser (445 nm) for material processing. Opt. Lett., 42, 2251(2017).
[2] S. Nagahara. Three primary color LD module. SID Symp. Digest Tech. Papers, 48, 80(2017).
[3] X. Liu, S. Yi, X. Zhou, Z. Fang, Z.-J. Qiu, L. Hu, C. Cong, L. Zheng, R. Liu, P. Tian. 34.5 m underwater optical wireless communication with 2.70 Gbps data rate based on a green laser diode with NRZ-OOK modulation. Opt. Express, 25, 27937(2017).
[4] X. Huang, F. Yang, J. Song. Hybrid LD and LED-based underwater optical communication: state-of-the-art, opportunities, challenges, and trends. Chin. Opt. Lett., 17, 100002(2019).
[5] L.-K. Chen, Y. Shao, R. Deng. Robust UOWC systems against bubble-induced impairments via transmit/receive diversities. Chin. Opt. Lett., 17, 100006(2019).
[6] H. König, M. Ali, W. Bergbauer, J. Brückner, G. Brüderl, C. Eichler, S. Gerhard, U. Heine, A. Lell, L. Naehle, M. Peter, J. Ristic, G. Rossbach, A. Somers, B. Stojetz, S. Tautz, J. Wagner, T. Wurm, U. Strauss, M. Baumann, A. Balck, V. Krause. Visible GaN laser diodes: from lowest thresholds to highest power levels. Proc. SPIE, 10939, 109390C(2019).
[7] E. Kioupakis, P. Rinke, C. G. Van de Walle. Determination of internal loss in nitride lasers from first principles. Appl. Phys. Express, 3, 082101(2010).
[8] E. Kioupakis, P. Rinke, A. Schleife, F. Bechstedt, C. G. Van de Walle. Free-carrier absorption in nitrides from first principles. Phys. Rev. B, 81, 241201(2010).
[9] D. Sizov, R. Bhat, C.-E. Zah. Optical absorption of Mg-doped layers and InGaN quantum wells on c-plane and semipolar GaN structures. J. Appl. Phys., 113, 203108(2013).
[10] L. Jiang, J. Liu, L. Zhang, B. Qiu, A. Tian, L. Hu, D. Li, S. Huang, W. Zhou, M. Ikeda, H. Yang. Suppression of substrate mode in GaN-based green laser diodes. Opt. Express, 28, 15497(2020).
[11] J. Simon, V. Protasenko, C. Lian, H. Xing, D. Jena. Polarization-induced hole doping in wide-band-gap uniaxial semiconductor heterostructures. Science, 327, 60(2010).
[12] S. Li, M. E. Ware, J. Wu, V. P. Kunets, M. Hawkridge, P. Minor, Z. Wang, Z. Wu, Y. Jiang, G. J. Salamo. Polarization doping: reservoir effects of the substrate in AlGaN graded layers. J. Appl. Phys., 112, 053711(2012).
[13] O. V. Khokhlev, K. A. Bulashevich, S. Y. Karpov. Polarization doping for III-nitride optoelectronics. Phys. Status Solidi A, 210, 1369(2013).
[14] Z. Zhang, M. Kushimoto, T. Sakai, N. Sugiyama, L. J. Schowalter, C. Sasaoka, H. Amano. A 271.8 nm deep-ultraviolet laser diode for room temperature operation. Appl. Phys. Express, 12, 124003(2019).
[15] K. Sato, S. Yasue, Y. Ogino, S. Tanaka, M. Iwaya, T. Takeuchi, S. Kamiyama, I. Akasaki. Light confinement and high current density in UVB laser diode structure using Al composition-graded p-AlGaN cladding layer. Appl. Phys. Lett., 114, 191103(2019).
[16] D. Zhao. III-nitride based ultraviolet laser diodes. J. Semicond., 40, 120402(2019).
[17] Z. Li, J. Liu, M. Feng, K. Zhou, S. Zhang, H. Wang, D. Li, L. Zhang, D. Zhao, D. Jiang, H. Wang, H. Yang. Suppression of thermal degradation of InGaN/GaN quantum wells in green laser diode structures during the epitaxial growth. Appl. Phys. Lett., 103, 152109(2013).
[18] A. Tian, J. Liu, M. Ikeda, S. Zhang, Z. Li, M. Feng, K. Zhou, D. Li, L. Zhang, P. Wen, F. Zhang, H. Yang. Conductivity enhancement in AlGaN:Mg by suppressing the incorporation of carbon impurity. Appl. Phys. Express, 8, 051001(2015).
[19] L. Hu, X. Ren, J. Liu, A. Tian, L. Jiang, S. Huang, W. Zhou, L. Zhang, H. Yang. High-power hybrid GaN-based green laser diodes with ITO cladding layer. Photon. Res., 8, 279(2020).
[20] T. Yasuda, K. Yagi, T. Suzuki, T. Nakashima, M. Watanabe, T. Takeuchi, M. Iwaya, S. Kamiyama, I. Akasaki. Investigations of polarization-induced hole accumulations and vertical hole conductions in GaN/AlGaN heterostructures. Jpn. J. Appl. Phys., 52, 08JJ05(2013).
[21] F. Bernardini, V. Fiorentini, D. Vanderbilt. Spontaneous polarization and piezoelectric constants of III-V nitrides. Phys. Rev. B, 56, R10024(1997).
[22] M. Asif Khan, A. Bhattarai, J. N. Kuznia, D. T. Olson. High electron mobility transistor based on a GaN-AlxGa1−xN heterojunction. Appl. Phys. Lett., 63, 1214(1993).
[23] K. Kim, W. R. L. Lambrecht, B. Segall. Elastic constants and related properties of tetrahedrally bonded BN, AlN, GaN, and InN. Phys. Rev. B, 53, 16310(1996).
[24] L. A. Coldren, S. W. Corzine, M. L. Mašanović. A phenomenological approach to diode lasers. Diode Lasers and Photonic Integrated Circuits(2012).
[25] M. Kawaguchi, O. Imafuji, S. Nozaki, H. Hagino, S. Takigawa, T. Katayama, T. Tanaka. Optical-loss suppressed InGaN laser diodes using undoped thick waveguide structure. Proc. SPIE, 9748, 974818(2016).
[26] H. Y. Ryu, K. H. Ha, J. K. Son, S. N. Lee, H. S. Paek, T. Jang, Y. J. Sung, K. S. Kim, H. K. Kim, Y. Park, O. H. Nam. Determination of internal parameters in blue InGaN laser diodes by the measurement of cavity-length dependent characteristics. Appl. Phys. Lett., 93, 011105(2008).
[27] D. J. Kunzmann, R. Kohlstedt, T. Uhlig, U. T. Schwarz. Critical discussion of the determination of internal losses in state-of-the-art (Al, In)GaN laser diodes. Proc. SPIE, 11280, 112800Y(2020).
[28] M. Kuramoto, C. Sasaoka, N. Futagawa, M. Nido, A. A. Yamaguchi. Reduction of internal loss and threshold current in a laser diode with a ridge by selective re-growth (RiS-LD). Phys. Status Solidi A, 192, 329(2002).
[29] Z. Zhang, M. Kushimoto, T. Sakai, N. Sugiyama, L. J. Schowalter, C. Sasaoka, H. Amano. Design and characterization of a low-optical-loss UV-C laser diode. Jpn. J. Appl. Phys., 59, 094001(2020).
[30] Z. Zhang, M. Kushimoto, M. Horita, N. Sugiyama, L. J. Schowalter, C. Sasaoka, H. Amano. Space charge profile study of AlGaN-based p-type distributed polarization doped claddings without impurity doping for UV-C laser diodes. Appl. Phys. Lett., 117, 152104(2020).
[31] K. Sato, S. Yasue, Y. Ogino, M. Iwaya, T. Takeuchi, S. Kamiyama, I. Akasaki. Analysis of spontaneous subpeak emission from the guide layers of the ultraviolet-B laser diode structure containing composition-graded p-AlGaN cladding layers. Phys. Status Solidi A, 217, 1900864(2020).
[32] T. Yasuda, K. Hayashi, S. Katsuno, T. Takeuchi, S. Kamiyama, M. Iwaya, I. Akasaki, H. Amano. Polarization dilution in a Ga-polar UV-LED to reduce the influence of polarization charges. Phys. Status Solidi A, 212, 920(2015).
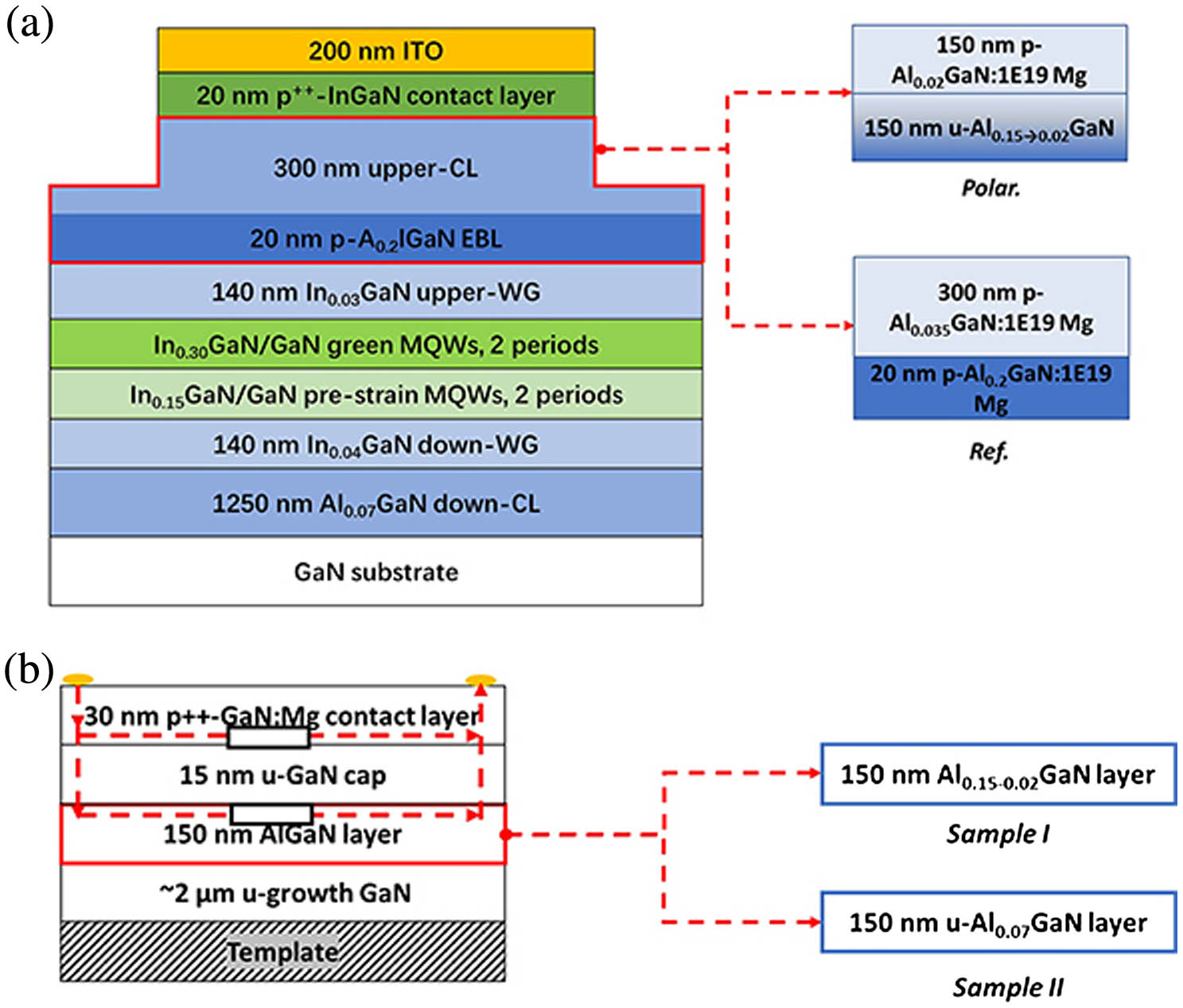
Set citation alerts for the article
Please enter your email address