
- Chinese Optics Letters
- Vol. 20, Issue 1, 013602 (2022)
Abstract
Keywords
1. Introduction
With the rapid development of terahertz (THz) science and technology, THz devices have aroused great interests[
In this work, a multifunctional THz metasurface device based on
2. Design and Result
Fig. 1(a) illustrates the sketch view of our proposed THz multifunctional metasurface. As depicted in Fig. 1(b), the unit cell consists of three layers: two L-shaped metal patterns, polyimide, and
L1 | L2 | W1 | W2 | G |
---|---|---|---|---|
125 | 155 | 25 | 30 | 60 |
Table 1. Parameters of EIT and Polarization Converter
Figure 1.(a) Sketch view and (b) unit cell of the proposed multifunctional device.
2.1. Dual-band EIT and linear to circular polarization converter
Figure 2(a) displays the scheme of the dual-band EIT and linear-to-circular polarization converter. Figure 2(b) shows the transmission of the proposed structure in Fig. 1 when the
Figure 2.(a) Scheme of the dual-band EIT and linear-to-circular polarization converter. (b) Transmission curves for the metasurface with the double L (black solid), the small L (blue dotted), and the large L (red dashed) shapes when VO2 is in the insulating state. The cross symbol denotes the fitted result based on the “two-particle” model. (c) The surface current distribution at the two transmission peaks.
To show the physical mechanism behind the dual-band EIT effect, the surface current distribution at the two transmission peaks
To further support the above assertion, the “two-particle” model is used to quantitatively describe the dual-band EIT effect. Here, two particles marked 1 and 2 represent the small and large L shape, respectively, which meet the following differential equation[
The simplified transmission for Eq. (1) can be obtained as
To verify the “two-particle” model of the dual-band EIT, two transparency windows are fitted separately and combined to form the complete curve. For the fitted analytical curve of two EIT bands, we get
The proposed unit cell structure behaves as a linear-to-circular polarization converter after the insulator-to-metal transition process. As demonstrated in Fig. 3(a), for the normal incident
Figure 3.(a) Reflection of Ryy and Rxy. (b) The phase and phase difference for the reflection of Ryy and Rxy. (c) The calculated ellipticity of the polarization conversion.
The amplitude and phase shift of the reflection are plotted in Figs. 3(a) and 3(b). The phase difference of two components (
2.2. Multifunctional metasurface antenna
Figure 4 shows the sketch map and basic unit cell of the multifunctional metasurface antenna with the parameters set in Table 2. On the top layer, two L shapes form the unit. The bottom layer is still made of
L1 | L2 | W1 | W2 | G |
---|---|---|---|---|
145 | 125 | 30 | 25 | 15 |
Table 2. Parameters of Planar-Array Antenna
Figure 4.Sketch map of the planar-array antenna and elements of each column.
Figure 5.(a) Transmission and (c) phase of cross polarization for VO2 in the insulating state. (b) Reflection and (d) phase of co-polarization for VO2 in the metallic state.
Considering the P-B phase, 60° phase difference for the six rotation elements could cover the 360° phase. The six rotation units are periodically arranged on the
Figure 6.(a) Three- and (b) two-dimensional radiation pattern of the reflected beam. (c) Three- and (d) two-dimensional radiation pattern of the transmitted beam for VO2 in the insulating state.
When
Figure 7.(a) Three- and (b) two-dimensional radiation pattern of the reflected beam when VO2 is in the metallic state.
3. Conclusion
To summarize, a multifunctional THz metasurface is presented based on the insulator-to-metal phase transition property of
By arranging the six unit cells with the metal pattern rotating 30°, a multifunctional metasurface antenna can be obtained. When
References
[1] B. Ferguson, X. Zhang. Materials for terahertz science and technology. Nat. Mater., 1, 26(2002).
[2] S. Katletz, M. Pfleger, H. Pühringer, M. Mikulics, N. Vieweg, O. Peters, B. Scherger, M. Scheller, M. Koch, K. Wiesauer. Polarization sensitive terahertz imaging: detection of birefringence and optical axis. Opt. Express, 20, 23025(2012).
[3] M. Tonouchi. Cutting-edge terahertz technology. Nat. Photonics, 1, 97(2007).
[4] R. A. Shelby, D. R. Smith, S. Schultz. Experimental verification of a negative index of refraction. Science, 292, 77(2001).
[5] N. Fang, H. Lee, C. Sun, X. Zhang. Sub-diffraction-limited optical imaging with a silver superlens. Science, 308, 534(2005).
[6] C. L. Holloway, E. F. Kuester, J. A. Gordon, J. O’Hara, J. Booth, D. R. Smith. An overview of the theory and applications of metasurfaces: the two-dimensional equivalents of metamaterials. IEEE Antennas Propag. Mag., 54, 10(2012).
[7] E. F. Kuester, M. A. Mohamed, M. Piket-May, C. L. Holloway. Averaged transition conditions for electromagnetic fields at a metafilm. IEEE Trans. Antenn. Propag., 51, 2641(2003).
[8] Y. Fan, Y. Qian, S. Yin, D. Li, M. Jiang, X. Lin, F. Hu. Multi-band tunable terahertz bandpass filter based on vanadium dioxide hybrid metamaterial. Mater. Res. Express, 6, 055809(2019).
[9] W. Liu, Z. Dai, J. Yang, Q. Sun, C. Gong, N. Zhang, K. Ueno, H. Misawa. Ultrabroad and angle tunable THz filter based on multiplexed metallic bar resonators. IEEE Photonics Technol. Lett., 30, 2103(2018).
[10] M. Masyukov, A. N. Grebenchukov, E. A. Litvinov, A. Baldycheva, A. V. Vozianova, M. K. Khodzitsky. Photo-tunable terahertz absorber based on intercalated few-layer graphene. J. Opt., 22, 095105(2020).
[11] N. I. Landy, S. Sajuyigbe, J. J. Mock, D. R. Smith, W. J. Padilla. Perfect metamaterial absorber. Phys. Rev. Lett., 100, 207402(2008).
[12] J. Hao, Y. Yuan, L. Ran, T. Jiang, J. Kong, C. Chan, L. Zhou. Manipulating electromagnetic wave polarizations by anisotropic metamaterials. Phys. Rev. Lett., 99, 063908(2007).
[13] Y. Wei, N. Panoiu. Polarization control using passive and active crossed graphene gratings. Opt. Express, 26, 1882(2018).
[14] Y. Jiang, L. Wang, J. Wang, C. N. Akwuruoha, W. Cao. Ultra-wideband high-efficiency reflective linear-to-circular polarization converter based on metasurface at terahertz frequencies. Opt. Express, 25, 27616(2017).
[15] H. Wang, Z. Zhang, K. Zhao, W. Liu, Y. Lu. Independent phase manipulation of co- and cross-polarizations with all-dielectric metasurface. Chin. Opt. Lett., 19, 053601(2021).
[16] J. Huang, T. Fu, H. Li, Z. Shou, X. Gao. A reconfigurable terahertz polarization converter based on metal–graphene hybrid metasurface. Chin. Opt. Lett., 18, 013102(2020).
[17] G. Zhou, P. Dai, J. Wu, B. Jin, Q. Wen, G. Zhu, Z. Shen, C. Zhang, L. Kang, W. Xu, J. Chen, P. Wu. Broadband and high modulation-depth THz modulator using low bias controlled VO2-integrated metasurface. Opt. Express, 25, 17322(2017).
[18] Z. Ma, S. M. Hanham, Y. Gong, M. Hong. All-dielectric reflective half-wave plate metasurface based on the anisotropic excitation of electric and magnetic dipole resonances. Opt. Lett., 43, 911(2018).
[19] L. Cong, N. Xu, J. Gu, R. Singh, J. Han, W. Zhang. Highly flexible broadband terahertz metamaterial quarter-wave plate. Laser Photon. Rev., 8, 626(2014).
[20] N. Yu, P. Genevet, M. A. Kats, F. Aieta, J.-P. Tetienne, F. Capasso, Z. Gaburro. Light propagation with phase discontinuities: generalized laws of reflection and refraction. Science, 334, 333(2011).
[21] Z. Song, J. Zhang. Achieving broadband absorption and polarization conversion in the same frequency band. Opt. Express, 28, 12487(2020).
[22] Y. Gao, X. Xiong, Z. Wang, F. Chen, R. Peng, M. Wang. Simultaneous generation of arbitrary assembly of polarization states with geometrical-scaling-induced phase modulation. Phys. Rev. X, 10, 031035(2020).
[23] J. Fan, Y. Cheng. Broadband high-efficiency cross-polarization conversion and multi-functional wavefront manipulation based on chiral structure metasurface for terahertz wave. J. Phys. D, 53, 025109(2020).
[24] Y. Li, J. Luo, X. Li, M. Pu, X. Luo. Switchable quarter-wave plate and half-wave plate based on phase-change metasurface. IEEE Photonics J., 12, 4600410(2020).
[25] Z. Wei, J. Jiang, Y. Jing, L. Shuang, L. Jiang. Functionality-switchable terahertz polarization converter based on a graphene-integrated planar metamaterial. OSA Continuum, 1, 124(2018).
[26] L. Peng, X. Li, X. Gao, X. Jiang, S. Li. Methodology for the design of a multi-functional device with switchable absorption and polarization conversion modes by graphene and metallic metasurfaces. Opt. Mater. Express, 9, 687(2019).
[27] H. Li, W. Xu, Q. Cui, Y. Wang, J. Yu. Theoretical design of a reconfigurable broadband integrated metamaterial terahertz device. Opt. Express, 28, 40060(2020).
[28] Q. Wen, H. Zhang, Q. Yang, Y. Xie, K. Chen, Y. Liu. Terahertz metamaterials with VO2 cut-wires for thermal tunability. Appl. Phys. Lett., 97, 021111(2010).
[29] L. Liu, L. Kang, T. S. Mayer, D. H. Werner. Hybrid metamaterials for electrically triggered multifunctional control. Nat. Commun., 7, 13236(2016).
[30] C. Zhang, G. Zhou, J. Wu, Y. Tang, P. Wu. Active control of terahertz waves using vanadium-dioxide-embedded metamaterials. Phys. Rev. Appl., 11, 054016(2019).
[31] J. Zhao, J. Song, Y. Zhou, Y. Liu, J. Zhou. Switching between the functions of half-wave plate and quarter-wave plate simply by using a vanadium dioxide film in a terahertz metamaterial. Chin. Phys. Lett., 37, 064204(2020).
[32] J. Luo, X. Shi, X. Luo, F. Hu, G. Li. Broadband switchable terahertz half-/quarter-wave plate based on metal-VO2 metamaterials. Opt. Express, 28, 30861(2020).
[33] H. Zhang, Y. Cao, Y. Liu, Y. Li. A novel graphene metamaterial design for tunable terahertz plasmon induced transparency by two bright mode coupling. Opt. Commun., 391, 9(2017).
[34] Y. Zhao, Q. Huang, H. Cai, X. Lin, H. He, H. Cheng, T. Ma, Y. Lu. Ultrafast control of slow light in THz electromagnetically induced transparency metasurfaces. Chin. Opt. Lett., 19, 073602(2021).
[35] W. Yu, H. Meng, Z. Chen, X. Li, X. Zhang, F. Wang, Z. Wei, C. Tan, X. Huang, S. Li. The bright–bright and bright–dark mode coupling-based planar metamaterial for plasmonic EIT-like effect. Opt. Commun., 414, 29(2018).
[36] L. Han, Q. Tan, Y. Gan, W. Zhang, J. Xiong. Polarization-insensitive classical electromagnetically induced transparency metamaterial with large group delay by Dirac semimetal. Results Phys., 19, 103377(2020).
[37] L. Zhu, Z. Xin, L. Dong, J. Guo, J. Xun, M. Zhong. Polarization-independent and angle-insensitive electromagnetically induced transparent (EIT) metamaterial based on bi-air-hole dielectric resonators. RSC Adv., 8, 27342(2018).
[38] F. Meng, Q. Wu, D. Erni, W. Ke, J. Lee. Polarization-independent metamaterial analog of electromagnetically induced transparency for a refractive-index-based sensor. IEEE Trans. Microw. Theory Tech., 60, 3013(2012).
[39] A. K. Fahad, C. Ruan, K. Chen. Dual-wide-band dual polarization terahertz linear to circular polarization converters based on bi-layered transmissive metasurfaces. Electronics, 8, 869(2019).
[40] W. S. L. Lee, R. T. Ako, M. X. Low, M. Bhaskaran, S. Sriram, C. Fumeaux, W. Withayachumnankul. Dielectric-resonator metasurfaces for broadband terahertz quarter- and half-wave mirrors. Opt. Express, 26, 14392(2018).
[41] Y. Hou, C. Zhang, C. Wang. High-efficiency and tunable terahertz linear-to-circular polarization converters based on all-dielectric metasurfaces. IEEE Access, 8, 140303(2020).
[42] L. Zhu, L. Dong, J. Guo, F. Meng, X. He, C. Zha, Q. Wu. Polarization conversion based on Mie-type electromagnetically induced transparency (EIT) effect in all-dielectric metasurface. Plasmonics, 13, 1971(2018).
[43] M. Amin, O. Siddiqui, M. Farhat. Linear and circular dichroism in graphene-based reflectors for polarization control. Phys. Rev. Appl., 13, 024046(2020).
[44] D. Yan, M. Meng, J. Li, J. Li, X. Li. Vanadium dioxide-assisted broadband absorption and linear-to-circular polarization conversion based on a single metasurface design for the terahertz wave. Opt. Express, 28, 29843(2020).
[45] J. Li, J. Yao. Manipulation of terahertz wave using coding Pancharatnam–Berry phase metasurface. IEEE Photonics J., 10, 5900512(2018).
[46] J. Li, Y. Zhang, J. Li, X. Yan, L. Liang, Z. Zhang, J. Huang, J. Li, Y. Yang, J. Yao. Amplitude modulation of anomalously reflected terahertz beams using all-optical active Pancharatnam–Berry coding metasurfaces. Nanoscale, 11, 5746(2019).
[47] Q. Zheng, Y. Li, J. Zhang, H. Ma, J. Wang, Y. Pang, Y. Han, S. Sui, Y. Shen, H. Chen, S. Qu. Wideband, wide-angle coding phase gradient metasurfaces based on Pancharatnam–Berry phase. Sci. Rep., 7, 43543(2017).
[48] B. Xiao, F. Lan, Z. Yang, P. Mazumder, J. Yin. Broadband and high-efficiency circular-polarized terahertz frequency scanning metasurface. Photonics&Electromagnetics Research Symposium—Fall (PIERS–Fall), 3229(2019).
[49] H. Zeng, F. Lan, Y. Zhang, T. Song, Z. Yang. Maximizing beam-scanning angle in an expected bandwidth based on terahertz metasurface with dual-mode resonance. Appl. Phys. Express, 12, 095501(2019).
[50] L. Wang, F. Lan, Y. Zhang, S. Liang, P. Mazumder. A fractional phase-coding strategy for terahertz beam patterning on digital metasurfaces. Opt. Express, 28, 6395(2020).
[51] S. Liu, T. Cui, L. Zhang, Q. Xu, Q. Wang, X. Wan, J. Gu, W. Tang, M. Qi, J. Han. Metasurfaces: convolution operations on coding metasurface to reach flexible and continuous controls of terahertz beams. Adv. Sci., 3, 1600156(2016).
[52] X. Fu, F. Yang, C. Liu, X. Wu, T. Cui. Terahertz beam steering technologies: from phased arrays to field-programmable metasurfaces. Adv. Opt. Mater., 8, 1900628(2020).
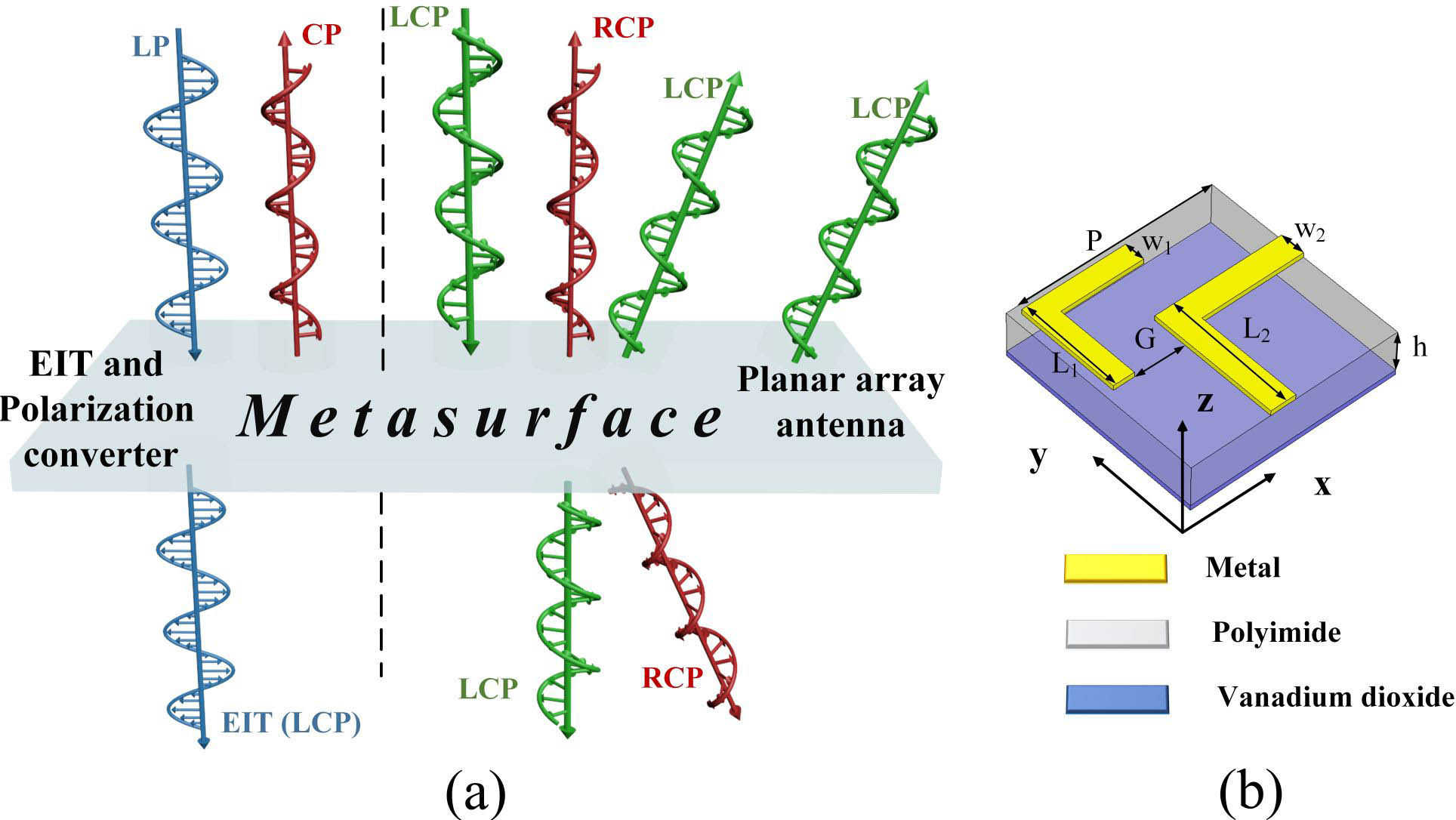
Set citation alerts for the article
Please enter your email address