Kunping Guo, Zhe Tang, Xingxing Chou, Saihu Pan, Chunchen Wan, Tao Xue, Liping Ding, Xiao Wang, Jin Huang, Fanghui Zhang, Bin Wei, "Printable organic light-emitting diodes for next-generation visible light communications: a review," Adv. Photon. Nexus 2, 044001 (2023)

Search by keywords or author
- Advanced Photonics Nexus
- Vol. 2, Issue 4, 044001 (2023)
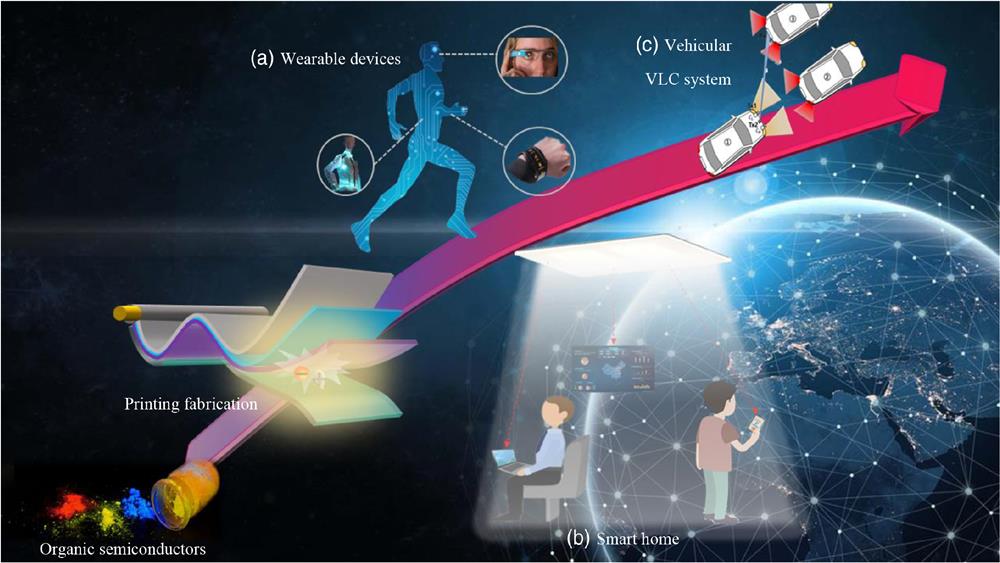
Fig. 1. Schematic illustration of OLED and OVLC applications. (a) Flexible and wearable OLED displays (Source: Fiere Electronics;14 Lifewire15). (b) Indoor environment for the VLC-based smart home. (c) Vehicular VLC system.
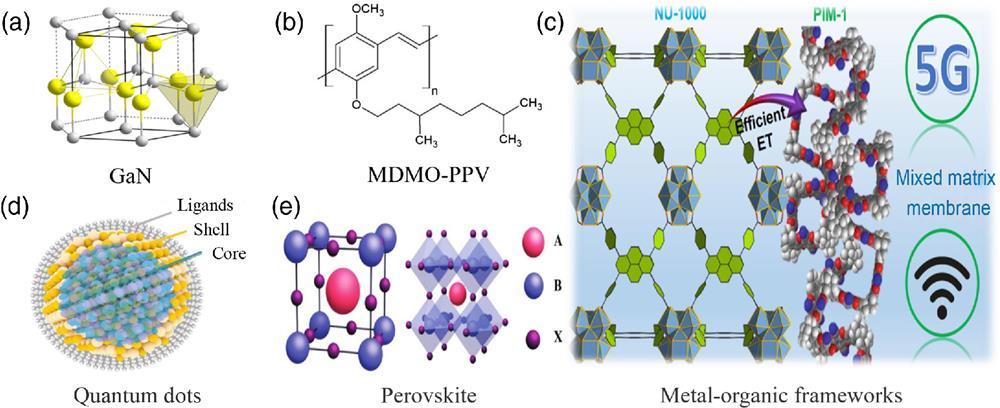
Fig. 2. (a) 3D crystal structure of GaN. (b) Chemical structure of MDMO-PPV. (c) Schematic of metal organic frameworks (MOFs) in mixed-matrix membranes for VLC. Reproduced and adapted with permission from Ref. 28, ©2022 American Chemical Society. (d) Schematic of a typical quantum dot (QD) (Source: AVS Forum29). (e) 3D crystal structure of a perovskite with the general formula . Reproduced and adapted with permission from Ref. 30, ©2017 Wiley-VCH Verlag GmbH & Co. KGaA, Weinheim.
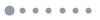
Fig. 3. (a) Schematic illustration of chitin nanofiber transparent paper for flexible green electronics. Adapted with permission from Ref. 63, ©2016 Wiley-VCH Verlag GmbH & Co. KGaA, Weinheim. (b) Transfer process of the OLED tattoo device and picture presenting working OLED tattoo transferred on an organic as well as a PVF nanosheet. Reproduced and adapted with permission from Ref. 64, ©2021 Wiley-VCH Verlag GmbH & Co. KGaA. (c) Schematic illustration of the photodynamic therapy (PDT) treatment principle and a photo of the wearable OLED-based PDT patch. Reproduced and adapted with permission from Ref. 65, ©2020 American Chemical Society.
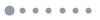
Fig. 4. (a) Schematic structure of the OLEDs. (b) Schematic illustration of phosphor-doped WOLED with conventional fluorescent host. (c) Schematic illustration of phosphor-doped WOLED with exciplex host. (d) Schematic diagram of all-TADF WOLEDs. (e) Schematic illustration of flexible WOLED structure based on PEAN and corresponding photograph of large-area WOLED with the size of . Reproduced and adapted with permission from Ref. 66, ©2014 American Chemical Society. (f) Schematic illustration of a tandem WOLED composed of orange and blue emitting units. Reproduced and adapted with permission from Ref. 67, ©2012 WILEY-VCH Verlag GmbH & Co. KGaA, Weinheim.
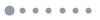
Fig. 5. (a) The number of scientific publications related to organic VLC published over the past 15 years as counted by the Web of Science, as of October 28, 2022. Search criteria were set to “visible light communication” and “organic.” (b) Trends of data rates versus publication years. (c) Photographs of the blue OLEDs and the corresponding images under a microscope. Reproduced and adapted with permission from Ref. 22, ©2020 Springer Nature.
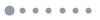
Fig. 6. (a) EQE-current density curves for the OLED. The electroluminescence (EL) spectrum is shown in the inset. (b) Eye diagrams characterized at with 1, 2.64, and 5 mA biases, as well as characterized at with 2.64 mA. (c) Block diagram of the experimental real-time VLC link. Reproduced and adapted with permission from Ref. 87, ©2020 Springer Nature.
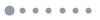
Fig. 7. (a) Schematic presenting the deposition process of inkjet printing with separator. photoluminescence microscopy imaging of QDs array with luminescent inks containing 100% and 90% cyclohexylbenzene; higher resolution photoluminescence microscopy imaging of a single dot corresponding to and . (b) Schematic view of the proposed process for slot-die roll coating. (c) Illustration of the top and front view of blade-coating process for fabricating multicolor polymer OLEDs. (d) Image of a completed 3D-printed OLED display. Reproduced and adapted with permission from: (a) Ref. 109, ©2017 American Chemical Society; (b) Ref. 100, ©2012 Springer Nature; (c) Ref. 110, © 2017 Wiley-VCH Verlag GmbH & Co. KGaA; (d) Ref. 111, © 2022 AAAS.
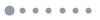
Fig. 8. (a) Diagram of the experimental setup used for the all-organic VLC system, adapted with permission from Ref. 98, © 2014 IEEE. (b) Photograph image of the main experimental setup of the all-organic flexible VLC link, adapted with permission from Ref. 99, ©2018 Multidisciplinary Digital Publishing Institute.
|
Table 1. Comparison of different solution-processed technologies.
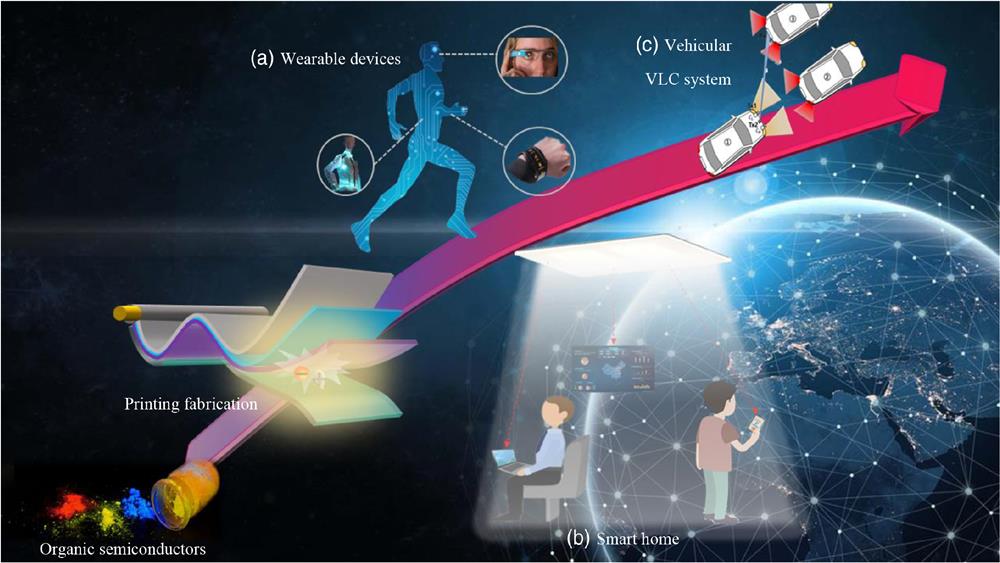
Set citation alerts for the article
Please enter your email address