Abstract
This study explores the epitaxial relationship and electrical properties of α-Ga2O3 thin films deposited on a-plane, m-plane, and r-plane sapphire substrates. We characterize the thin films by X-ray diffraction and Raman spectroscopy, and elucidate thin film epitaxial relationships with the underlying sapphire substrates. The oxygen vacancy concentration of α-Ga2O3 thin films on m-plane and r-plane sapphire substrates are higher than α-Ga2O3 thin film on a-plane sapphire substrates. All three thin films have a high transmission of over 80% in the visible and near-ultraviolet regions, and their optical bandgaps stay around 5.02–5.16 eV. Hall measurements show that the α-Ga2O3 thin film grown on r-plane sapphire has the highest conductivity of 2.71 S/cm, which is at least 90 times higher than the film on a-plane sapphire. A similar orientation-dependence is seen in their activation energy as revealed by temperature-dependent conductivity measurements, with 0.266, 0.079, and 0.075 eV for the film on a-, m-, r-plane, respectively. The origin of the distinct transport behavior of films on differently oriented substrates is suggested to relate with the distinct evolution of oxygen vacancies at differently oriented substrates. This study provides insights for the substrate selection when growing α-Ga2O3 films with tunable transport properties.1. Introduction
As an emerging third-generation semiconductor material, gallium oxide (Ga2O3) has superior properties such as wide band gap, high breakdown electric field, and strong radiation resistance, which can more effectively serve the emerging needs of power electronic device development, such as Schottky barrier diodes (SBDs)[1,2], heterojunction diodes[3,4], metal-oxide semiconductor field-effect transistors (MOSFETs)[5,6], etc. compared with Si, SiC and GaN[5,7-9]. It has a wide range of application prospects in the field of advanced information technology and new energy.
Ga2O3 has five crystalline phases:α,β,δ,γ andε. Among them, the monoclinic structureβ-Ga2O3 is a thermally stable phase at high temperature, and the other four phases are all metastable crystalline phases[10-16]. But, due to the lack of symmetry of monoclinicβ-Ga2O3 compared with the hexagonal system, there is a problem of "twisting" in the heteroepitaxial, which is a major difficulty in the crystal quality of heteroepitaxial thin films[17]. Compared withβ-Ga2O3, the corundom structureα-Ga2O3 is one of the metastable phases and has a wider band gap and higher breakdown field strength, making it more suitable for the fabrication of power electronic devices[18]. Bothα-Ga2O3 (a = 4.98 Å,c = 13.43 Å)[19] and sapphire (a = 4.76 Å,c = 12.99 Å) (PDF: 46-1212) belong to a corundum structure, and the lattice mismatch between them is small. Therefore, sapphire is well suited as a substrate for epitaxialα-Ga2O3. We have mainly selected sapphire substrates with three different crystal orientations: a-plane (
), m-plane (
) and r-plane (
). The study of the electrical properties ofα-Ga2O3 thin films is an important basis for the fabrication of high-performance devices. However, the effect of substrate crystal orientation on the thin film properties remains contentious, including the crystalline quality and the electrical properties of the thin films[7,20-23]. The anisotropy of the electrical properties of Ga2O3 may be follows from the anisotropy of the long-range electron–phonon interaction, as well as from the conduction band anisotropy[24]. On the other hand, the defects also play a crucial role in the electrical properties[25]. Therefore, there is a significant need to investigate the effect of substrate crystal orientation on the heteroepitaxial and electrical properties of the films.
The main methods currently used to grow Ga2O3 films are radio frequency magnetron sputtering (RFMS)[25,26], metal organic chemical vapor deposition (MOCVD)[14,27-29], molecular beam epitaxy (MBE)[7,8]. Compared to the above methods, pulsed laser deposition (PLD) has the following outstanding advantages: fast film growth, easy doping, easy preparation of films with the same stoichiometric ratio as the target, easy adjustment of the growth process[10,11,13,30].
In this work, we have systematically investigated the epitaxial relationships and the differences in electrical properties of epitaxialα-Ga2O3 films on a-plane, m-plane, and r-plane sapphire substrates using the PLD technique. We found that the epitaxial films have the same corundum structure and the growth orientation as the substrate, respectively. The conductivity shows an increasing trend from a-plane to m-plane to r-plane, which may be related to oxygen vacancy.
2. Experimental
The 1% Sn-dopedα-Ga2O3 thin films were deposited with the same growth condition on a-plane, m-plane, and r-plane sapphire substrates by PLD (SKY Technology Development Co., Ltd. Chinese Academy of Sciences), at about 800 °C, the oxygen pressure was fixed at 0.1 mTorr. The epitaxial growth relationship and crystalline structure ofα-Ga2O3 film were analyzed by X-ray diffraction (XRD, D8 Discover). The structure of theα-Ga2O3 thin films was also confirmed by Raman spectroscopy (Renishaw inVia Reflex) at room temperature using a laser beam of 532 nm. Film transmission in the range of 200–800 nm was measured by a UV-visible spectrophotometer (UV-vis, Lambda 1050). The elemental composition and chemical state were characterized by X-ray photoelectron spectroscopy (XPS, Kratos AXIS SUPRA) using Mg Kα (hν = 1253.6 eV) as the excitation source. The electrode spacing and effective electrode area were controlled using a direct-write optical lithography machine (MicroWriter ML3) and a lift-off process. The electrical properties at room temperature and varies temperatures were measured in the Hall measurement system (HL-5500PC) with a 0.5 T magnet, and in a semiconductor parameter analyzer (Keithley 4200-SCS), respectively.
3. Results and discussion
The schematic diagram of different crystal planes in the corundom structure are shown inFig. 1(a). The lattice mismatch (δ) is calculated according to the following equation:δ = (
e –
s)/
s. Here,
e and
s indicate the lattice constants of epitaxial thin films and substrates, respectively. The lattice mismatches between different crystal planesα-Ga2O3 films and corresponding sapphire substrates are shown inFig. 1(b). It is easy to find that the lattice mismatch between r-plane sapphire and r-planeα-Ga2O3 of the long side is larger than that of the a-plane and m-plane, which may be the reason why it is more difficult to epitaxy Ga2O3 on r-plane sapphire.
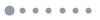
Figure 1.(Color online) (a) Schematic diagram of various crystal planes in the corundom structure. (b) The lattice mismatch between different crystal planes α-Ga2O3 films and corresponding sapphire substrates.
We determine the crystalline phase of Ga2O3 using XRD and Raman scattering. As shown inFig. 2(a), the (
) and (
) diffraction peaks ofα-Ga2O3 are accompanied by (
) and (
) diffraction peaks of a-plane sapphire, the (
) diffraction peak ofα-Ga2O3 are accompanied by (30
0) diffraction peak of m-plane sapphire, and the (
), (
) and (
) diffraction peaks ofα-Ga2O3 are accompanied by (
), (
) and (
) diffraction peaks of r-plane sapphire. The structure ofα-Ga2O3 thin films is also confirmed by Raman scattering. The XRDθ–2θ scan patterns of the above three samples all show that the crystal orientation of theα-Ga2O3 films is almost consistent with the sapphire substrate.
Substrate | Thickness (nm) | σ (S/cm) | μ (cm2/(V·s)) | n (1017 cm−3) |
---|
a-sapphire | 302 | Exceed test limit (<0.03) | – | – |
m-sapphire | 280 | 0.29 | 12.2 | 1.45 |
r-sapphire | 273 | 2.71 | 1.35 | 125 |
Table 1. Film thicknesses, conductivities, mobilities, and carrier concentrations for α-Ga2O3 thin films grown on a-, c- and r-plane sapphire substrates.
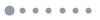
Figure 2.(Color online) (a) XRDθ–2θ full scans ofα-Ga2O3 thin films grown on a-plane, m-plane, and r-plane sapphire substrates. (b) XRD
-scans of (
) plane, (
) plane, and (
) plane of correspondingα-Ga2O3 films and the underlying sapphire, respectively. (c) Raman scattering spectra ofα-Ga2O3 thin films grown on a-plane, m-plane, and r-plane sapphire substrates. (d) XRD rocking curves around theα-Ga2O3 (
) peak grown on a-plane sapphire,α-Ga2O3 (
) peak grown on m-plane sapphire, andα-Ga2O3 (
) peak grown on r-plane sapphire.
XRD
-scan mode measurements are used to further determine the in-plane epitaxial growth relationship ofα-Ga2O3 film relative to sapphire substrates. As shown inFig. 2(b), the characteristic (
) of a-planeα-Ga2O3 thin film and (
) of a-plane sapphire substrate appear at the same rotational angle of
, suggesting that theα-Ga2O3 thin film has the same corundom structure as the sapphire substrate. The (
) of the m-planeα-Ga2O3 thin film and sapphire substrate, and the (
) of r-planeα-Ga2O3 thin film and sapphire substrate show similar results to those above.
Fig. 2(c) shows the Raman shift ofα-Ga2O3 thin films grown on a-, m-, r-plane sapphire substrates. The Raman peaks belonging toα-Ga2O3 thin film are mainly located at 430.4, 575.8 cm−1, which is consistent with reported values[31,32]. The Raman results are consistent with the XRD results. The XRD rocking curves of a-planeα-Ga2O3 (
) peak, m-planeα-Ga2O3 (30
0) peak, and r-planeα-Ga2O3 (
) peak are shown inFig. 2(d). The fullwidth at half maximum (FWHM) of theω-scan rocking curves of (
) peak, (30
0) peak, and (
) peak are 0.34°, 0.57°, and 0.85°, respectively. This indicates that the crystalline quality of a-planeα-Ga2O3 is greater at the a-plane than m-plane and r-plane, which is affected by multiple factors, including the different crystalline quality of the substrate with different orientations, the lattice mismatch, and the nucleation kinetics for different orientations[17,33,34].
Based on the above test results, it is not difficult to deduce the growth behaviors ofα-Ga2O3 thin films grown on differently-oriented sapphire substrates. As shown inFig. 3, the unit cell arrangement ofα-Ga2O3 thin films is almost the same as that of the corresponding sapphire substrates.
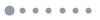
Figure 3.(Color online) Schematic of growth relation of α-Ga2O3 grown on (a) a-plane, (b) m-plane, and (c) r-plane sapphire substrates by PLD.
Then, we probed the defect density and distribution of differently-orientedα-Ga2O3 films using XPS.Fig. 4 shows the O 1s core level spectra of various planeα-Ga2O3 thin films. We calibrate the data with a standard binding energy of 284.8 eV for the C 1s peak[35]. The XPS O 1s core level spectra of the films are divided into two individual peaks and well fitted with the sum Gaussian functions. The positions of the two peaks correspond to lattice oxygen of Ga2O3 (OI) and oxygen vacancies (OII), respectively[26]. We can use OII/(OI+OII) to reflect the concentration of oxygen vacancies. The oxygen vacancy concentration of the a-plane, m-plane, and r-planeα-Ga2O3 are 11.42%, 20.31%, and 19.29%, respectively.
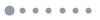
Figure 4.(Color online) XPS spectra of O 1s peak of α-Ga2O3 films grown on (a) a-plane, (b) m-plane, and (c) r-plane sapphire substrates.
Furthermore, the UV-vis transmission spectra for as grownα-Ga2O3 epitaxial films on differently-oriented sapphires are illustrated inFig. 5(a). Both films have high transparency (>80%) in the visible and near-UV regions. As shown inFig. 5(b), the optical band gap (Eg) of theα-Ga2O3 films can be evaluated using the plot of (αhν)2 vshν, whereα is the absorption coefficient andhν is the photon energy. a-plane, m-plane, and r-planeα-Ga2O3 films have an estimated bandgap of 5.16, 5.12, and 5.02 eV, respectively.
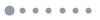
Figure 5.(Color online) (a) Transmittance spectra and (b) Tauc plot of the a-plane, m-plane, and r-plane α-Ga2O3 thin films grown on various plane sapphire substrates.
In order to explore the electrical transport properties of differently-orientedα-Ga2O3 thin films, Hall measurement is performed in a conventional van der Pauw geometry.Table 1 shows the results of Hall measurements. The conductivity (σ) of theα-Ga2O3 thin film epitaxial on a-plane sapphire exceeds the measurement capacity of the Hall tester. Forα-Ga2O3 thin film epitaxial on m-plane sapphire, Hall measurements indicate that the film is n-type conductive with a high conductivity of 0.29 S/cm, a mobility (μ) of 12.2 cm2/(V·s) and a carrier concentration (n) of 1.45 × 1017 cm−3. While theα-Ga2O3 thin film epitaxial on r-plane sapphire has a higher conductivity of 2.71 S/cm, a mobility of 1.35 cm2/(V·s) and a high carrier concentration of 1.25 × 1019 cm−3.
The temperature dependence of the resistance in the range from 295 to 473 K for the Sn-dopedα-Ga2O3 thin films are shown inFig. 6. The activation energy (Ea) is derived from the linear fit of the ln(R)–T plot usingR ∝ exp (Ea/kBT), whereR is the resistance,kB is the Boltzmann constant, andT is the temperature[10,36]. The estimated activation energy forα-Ga2O3 epitaxial on a-plane, m-plane and r-plane sapphire substrates are 0.266, 0.079, and 0.075 eV, respectively. The value of activation energy is used to quantify the thermal ionization of defect states[28].
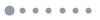
Figure 6.(Color online) Fitting plots of temperature-dependent resistance deriving the activation energy for a-plane, m-plane, and r-plane α-Ga2O3 thin films.
Theα-Ga2O3 thin films on m-plane and r-plane sapphire substrates are far more conducting thanα-Ga2O3 thin film on a-plane sapphire substrate under similar thin film growth conditions. It is interesting that the oxygen vacancy concentration ofα-Ga2O3 thin films on m-plane and r-plane sapphire substrates are also higher thanα-Ga2O3 thin film on a-plane sapphire substrates. It is speculated that the conductivity behavior ofα-Ga2O3 thin films may be related to the oxygen vacancy concentration, and the formation energy could strongly depend on the lattice orientation, thus leading to drastical differences in the dopant activation and carrier transport[29,37,38]. Further studies on the mechanism of the effect of anisotropy and crystallinity on electrical conductivity are expected.
4. Conclusions
We have investigated the Sn-dopedα-Ga2O3 thin films deposited on a-plane, m-plane, and r-plane sapphire substrates by PLD. Due to the same corundum structure, the out-of-plane and in-plane alignment ofα-Ga2O3 films is almost identical to that of the corresponding oriented sapphire substrate. The FWHMs ofω-scan rocking curves of the a-plane, m-plane, and r-planeα-Ga2O3 thin films are 0.34°, 0.57°, and 0.85°, respectively. The r-planeα-Ga2O3 thin film has the highest conductivity, the m-planeα-Ga2O3 thin film the second, and the a-planeα-Ga2O3 thin film dramatically more insulating. A similar trend is also observed in their activation energy. This could be understood by that the orientation difference leads to the concentration variation of oxygen defects in differently orientedα-Ga2O3 thin films, further causing differences in the electrical properties of the thin films.
References
[1] K Konishi, K Goto, H Murakami et al. 1-kV vertical Ga2O3 field-plated Schottky barrier diodes. Appl Phys Lett, 110, 103506(2017).
[2] Z Z Hu, H Zhou, Q Feng et al. Field-plated lateral β-Ga2O3 Schottky barrier diode with high reverse blocking voltage of more than 3 kV and high DC power figure-of-merit of 500 MW/cm2. IEEE Electron Device Lett, 39, 1564(2018).
[3] W B Hao, Q M He, X Z Zhou et al. 2.6 kV NiO/Ga2O3 heterojunction diode with superior high-temperature voltage blocking capability. 2022 IEEE 34th International Symposium on Power Semiconductor Devices and ICs (ISPSD), 105(2022).
[4] H H Gong, X H Chen, Y Xu et al. A 1.86-kV double-layered NiO/β-Ga2O3 vertical p–n heterojunction diode. Appl Phys Lett, 117, 022104(2020).
[5] X Z Zhou, Y J Ma, G W Xu et al. Enhancement-mode β-Ga2O3 U-shaped gate trench vertical MOSFET realized by oxygen annealing. Appl Phys Lett, 121, 223501(2022).
[6] N Moser, J McCandless, A Crespo et al. Ge-doped β-Ga2O3 MOSFETs. IEEE Electron Device Lett, 38, 775(2017).
[7] R Jinno, C S Chang, T Onuma et al. Crystal orientation dictated epitaxy of ultrawide-bandgap 5.4- to 8.6-eV α-(AlGa)2O3 on m-plane sapphire. Sci Adv, 7, eabd5891(2021).
[8] M Kracht, A Karg, M Feneberg et al. Anisotropic optical properties of metastable (011-2) α–Ga2O3 grown by plasma-assisted molecular beam epitaxy. Phys Rev Applied, 10, 024047(2018).
[9] Z Y Wu, Z X Jiang, P Y Song et al. Nanowire-seeded growth of single-crystalline (010) β-Ga2 O3 nanosheets with high field-effect electron mobility and on/off current ratio. Small, 15, e1900580(2019).
[10] W R Zhang, J G Zhang, L Chen et al. Non-equilibrium epitaxy of metastable polymorphs of ultrawide-bandgap gallium oxide. Appl Phys Lett, 120, 072101(2022).
[11] J G Zhang, W Wang, S M Wu et al. Exploratory phase stabilization in heteroepitaxial gallium oxide films by pulsed laser deposition. J Alloys Compd, 935, 168123(2023).
[12] C Wu, D Y Guo, L Y Zhang et al. Systematic investigation of the growth kinetics of β-Ga2O3 epilayer by plasma enhanced chemical vapor deposition. Appl Phys Lett, 116, 072102(2020).
[13] W Wang, Q L Yuan, D Y Han et al. High-temperature deep ultraviolet photodetector based on a crystalline Ga2O3-diamond heterostructure. IEEE Electron Device Lett, 43, 2121(2022).
[14] Y B Zhang, J Zheng, P P Ma et al. Growth and characterization of β-Ga2O3 thin films grown on off-angled Al2O3 substrates by metal-organic chemical vapor deposition. J Semicond, 43, 092801(2022).
[15] H D Sun, K H Li, C G Torres Castanedo et al. HCl flow-induced phase change of α-, β-, and ε-Ga2O3 films grown by MOCVD. Cryst Growth Des, 18, 2370(2018).
[16] X H Hou, H D Sun, S B Long et al. Ultrahigh-performance solar-blind photodetector based on α-phase-dominated Ga2O3 film with record low dark current of 81 fA. IEEE Electron Device Lett, 40, 1483(2019).
[17] S Nakagomi, Y Kokubun. Crystal orientation of β-Ga2O3 thin films formed on c-plane and a-plane sapphire substrate. J Cryst Growth, 349, 12(2012).
[18] X H Chen, F F Ren, S L Gu et al. Review of gallium-oxide-based solar-blind ultraviolet photodetectors. Photon Res, 7, 381(2019).
[19] M Marezio, J P Remeika. Bond lengths in the α-Ga2O3 structure and the high-pressure phase of Ga2−xFexO3. J Chem Phys, 46, 1862(1967).
[20] S Nakagomi, S Kaneko, Y Kokubun. Crystal orientations of β-Ga2O3 thin films formed on m-plane and r-plane sapphire substrates. Phys Status Solidi B, 252, 612(2015).
[21] Y L Cheng, Y Xu, Z Li et al. Heteroepitaxial growth of α-Ga2O3 thin films on a-, c- and r-plane sapphire substrates by low-cost mist-CVD method. J Alloys Compd, 831, 154776(2020).
[22] H Z Hu, C Wu, N Zhao et al. Epitaxial growth and solar-blind photoelectric characteristic of Ga2O3 film on various oriented sapphire substrates by plasma-enhanced chemical vapor deposition. Phys Status Solidi A, 218, 2100076(2021).
[23] E Chikoidze, D J Rogers, F H Teherani et al. Puzzling robust 2D metallic conductivity in undoped β-Ga2O3 thin films. Mater Today Phys, 8, 10(2019).
[24] K Ghosh, U Singisetti. Electron mobility in monoclinic β-Ga2O3—Effect of plasmon-phonon coupling, anisotropy, and confinement. J Mater Res, 32, 4142(2017).
[25] X Hou, X Zhao, Y Zhang et al. High-performance harsh-environment-resistant GaOX solar-blind photodetectors via defect and doping engineering. Adv Mater, 34, e2106923(2022).
[26] T Zhang, D G Guan, N T Liu et al. Room temperature fabrication and post-annealing treatment of amorphous Ga2O3 photodetectors for deep-ultraviolet light detection. Appl Phys Express, 15, 022007(2022).
[27] X Q Xiang, L H Li, C Chen et al. Unintentional doping effect in Si-doped MOCVD β-Ga2O3 films: Shallow donor states. Sci China Mater, 66, 748(2023).
[28] Y Qin, L H Li, X L Zhao et al. Metal–semiconductor–metal ε-Ga2O3 solar-blind photodetectors with a record-high responsivity rejection ratio and their gain mechanism. ACS Photonics, 7, 812(2020).
[29] A Waseem, Z J Ren, H C Huang et al. A review of recent progress in β-Ga2O3 epitaxial growth: Effect of substrate orientation and precursors in metal–organic chemical vapor deposition. Phys Status Solidi A, 2200616(2022).
[30] N T Liu, T Zhang, L Chen et al. Fast-response amorphous Ga2O3 solar-blind ultraviolet photodetectors tuned by a polar AlN template. IEEE Electron Device Lett, 43, 68(2022).
[31] R Cuscó, N Domènech-Amador, T Hatakeyama et al. Lattice dynamics of a mist-chemical vapor deposition-grown corundum-like Ga2O3 single crystal. J Appl Phys, 117, 185706(2015).
[32] T C Ma, X H Chen, F F Ren et al. Heteroepitaxial growth of thick α-Ga2O3 film on sapphire (0001) by MIST-CVD technique. J Semicond, 40, 012804(2019).
[33] P Mazzolini, A Falkenstein, C Wouters et al. Substrate-orientation dependence of β-Ga2O3 (100), (010), (001), and (-201) homoepitaxy by indium-mediated metal-exchange catalyzed molecular beam epitaxy (MEXCAT-MBE). APL Mater, 8, 011107(2020).
[34] C C Yen, T M Huang, P W Chen et al. Role of interfacial oxide in the preferred orientation of Ga2O3 on Si for deep ultraviolet photodetectors. ACS Omega, 6, 29149(2021).
[35] B R Tak, S Dewan, A Goyal et al. Point defects induced work function modulation of β-Ga2O3. Appl Surf Sci, 465, 973(2019).
[36] A Miller, E Abrahams. Impurity conduction at low concentrations. Phys Rev, 120, 745(1960).
[37] Z Hajnal, J Miró, G Kiss et al. Role of oxygen vacancy defect states in the n-type conduction of β-Ga2O3. J Appl Phys, 86, 3792(1999).
[38] J Y Zhang, J L Shi, D C Qi et al. Recent progress on the electronic structure, defect, and doping properties of Ga2O3. APL Mater, 8, 020906(2020).