Jingshu Guo, Laiwen Yu, Hengtai Xiang, Yuqi Zhao, Chaoyue Liu, Daoxin Dai, "Realization of advanced passive silicon photonic devices with subwavelength grating structures developed by efficient inverse design," Adv. Photon. Nexus 2, 026005 (2023)

Search by keywords or author
- Advanced Photonics Nexus
- Vol. 2, Issue 2, 026005 (2023)
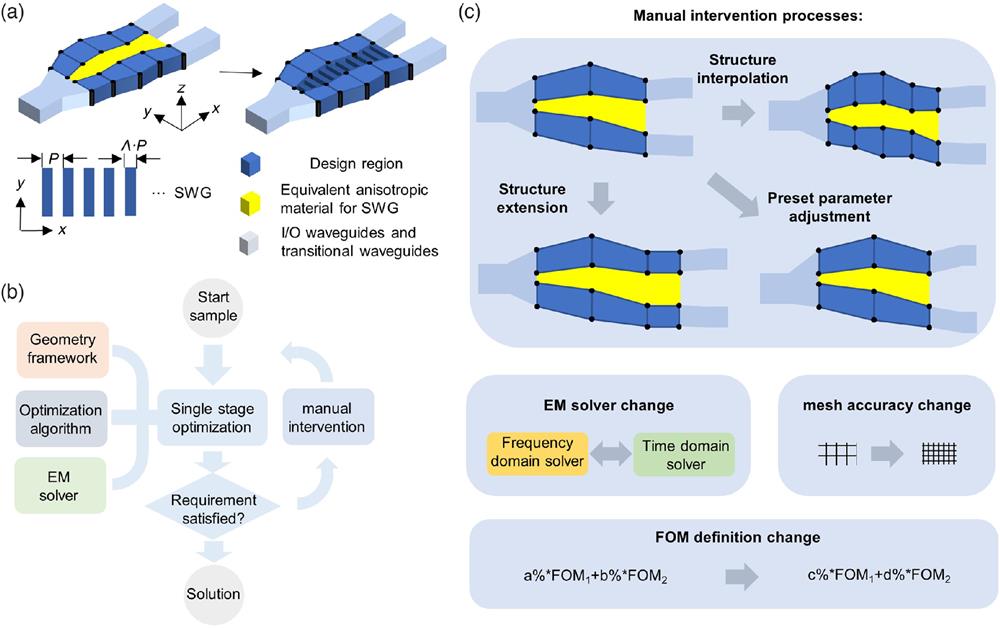
Fig. 1. Proposed inverse design strategy for passive photonic devices. (a) The design framework is demonstrated by an example of a photonic device with one input port and two output ports. Here, the SWG has a period of and a fill factor of . (b) Design flow chart with manual interventions. (c) Typical manual intervention operations.

Fig. 2. Inverse design of a six-channel mode (de)multiplexer on silicon. (a) Top view ( plane), 3D view, and cross-sectional view ( plane) at the interface between section and section (not to scale). (b) FOM and the simulation time cost as functions of the iteration generation for air-slot and -slot devices. Inset: the milestone structures in the optimization flow. (c) and (d) The 3D FDTD simulation performance of the designed -filled mode (de)multiplexer including the transmissions (c) and the light propagation fields for different input modes at 1550 nm (d). Modes #1 to #6 are, respectively, the to modes supported by the bus waveguides (Port #1), and modes #7 to #12 are, respectively, the mode of Ports #2 to #7. Here the silicon photonic waveguides with a 220-nm-thick silicon core and a upper-cladding are used.
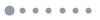
Fig. 3. Fabricated devices and measured results. (a) Microscope picture for the fabricated silicon PIC consisting of a pair of mode (de)multiplexers with six input ports ( ) and six output ports ( ). (b) SEM image of a mode (de)multiplexer. (c) Normalized transmissions of different port pairs.
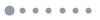
Fig. 4. Inverse design of a 90 deg hybrid on silicon. (a) The 3D schematic diagram. LO, local oscillator. (b)–(f) The simulated performances of the final design ( slot device): the simulated light propagation fields with varied phase difference between Ports 2 and 1 at (b) 1550 nm, (c) the transmissions, (d) CMMRs, (e) the Els, and (f) phase errors (f). Here the transmission is given by the parameter (i.e., channel is given by ). CMMR, common mode rejection ratio.
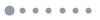
Fig. 5. Experimental results of the fabricated 90 deg hybrid. (a) Measured transmissions. (b) Measured CMMRs. (c) Measured ELs. (d) The port-to-port optical transmission are spectra measured by the phase-test PIC. (e) Phase error extracted from the measured results of the phase-test PIC.
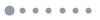
Fig. 6. The inverse-designed two-channel wavelength multiplexer on silicon. (a) 3D schematic diagram. (b) Simulated light propagation in the designed devices. (c) Calculated transmissions. (d) Measured transmissions. Inset: SEM image of the fabricated wavelength demultiplexer.
|
Table 1. Summary of the state-of-the-art mode (de)multiplexers, 90 deg hybrids, and wavelength (de)multiplexers on silicon.
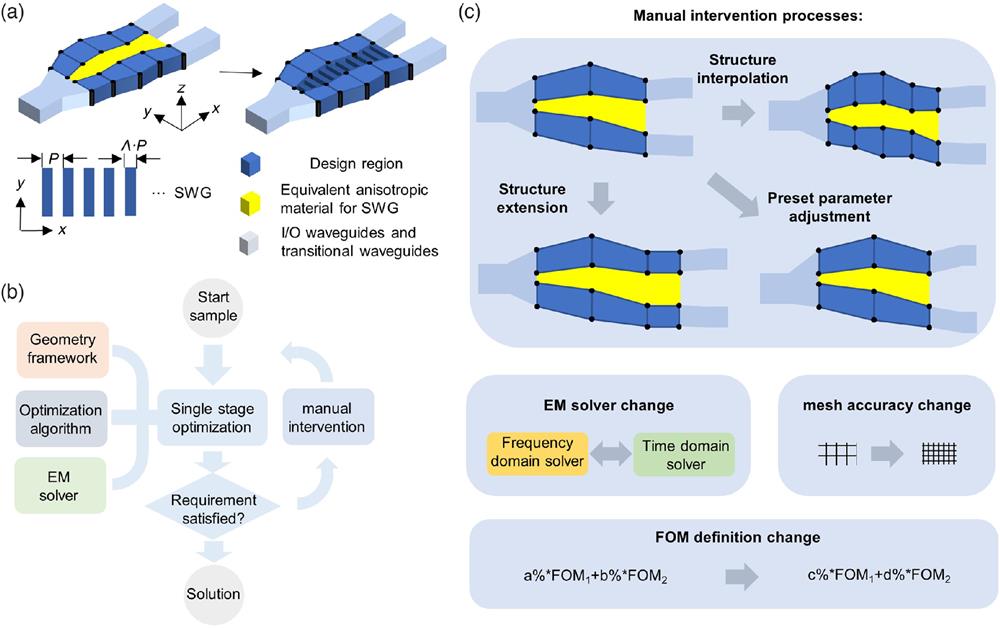
Set citation alerts for the article
Please enter your email address