Muye Li, Xuezong Yang, Yuxiang Sun, Zhenxu Bai, Yan Feng. Single-frequency continuous-wave diamond Raman laser (Invited)[J]. Infrared and Laser Engineering, 2022, 51(6): 20210970

Search by keywords or author
- Infrared and Laser Engineering
- Vol. 51, Issue 6, 20210970 (2022)
![[in Chinese]](/richHtml/irla/2022/51/6/20210970/img_1.jpg)
Fig. 1. [in Chinese]
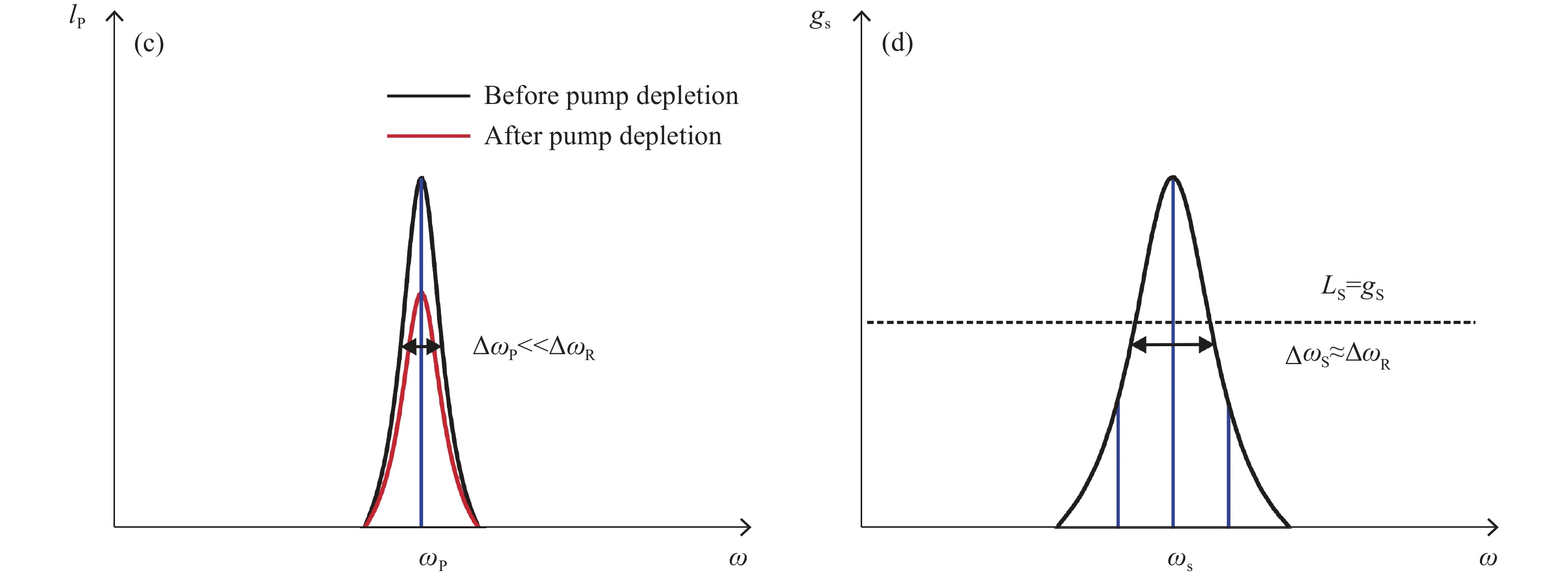
Fig. 1. Pump spectrum and Stokes gain spectrum when pump linewidth is longer ((a), (b)) and shorter ((c), (d)) than the Raman gain linewidth
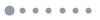
Fig. 2. Response of phonon field amplitude in Raman laser and the inversion density in a conventional laser when the standing-wave cavity is resonating
![Schematic of the Raman oscillator based on the free spatial hole burning property in SRS. IM: input mirror, OC: output coupler, LPF: long-pass filter, L1 and L2: lenses[26]](/Images/icon/loading.gif)
Fig. 3. Schematic of the Raman oscillator based on the free spatial hole burning property in SRS. IM: input mirror, OC: output coupler, LPF: long-pass filter, L1 and L2: lenses[26]
![Scanning Fabry-Pérot interferometer traces of the Raman laser emission[26]](/Images/icon/loading.gif)
Fig. 4. Scanning Fabry-Pérot interferometer traces of the Raman laser emission[26]
![Schemematic layout of the Raman laser by using H-C locking strategy, BS: beam sampler, HWP : half- wave plate, FL: focusing lens, IC: input coupler, OC: output coupler, PZT: piezoelectric translation stage, DM: dichroic mirror, QWP: quarter-wave plate, PBS: polarizing beam splitter, PD1 and PD2: photodetectors[27]](/Images/icon/loading.gif)
Fig. 5. Schemematic layout of the Raman laser by using H-C locking strategy, BS: beam sampler, HWP : half- wave plate, FL: focusing lens, IC: input coupler, OC: output coupler, PZT: piezoelectric translation stage, DM: dichroic mirror, QWP: quarter-wave plate, PBS: polarizing beam splitter, PD1 and PD2: photodetectors[27]
![Schematic layout of resonant pumping Raman laser by direction control of field in the ring cavity. The Pump source is the SolsTis Ti:Sapphire laser from M Squared Lasers Ltd. HR: high reflector; PR: partial reflector; IC/OC: input/output coupler; DM: dichroic mirror, HR at Stokes, HT at pump; BS: uncoated beam sampler; BM: beam dump; λ∕2: half-wave plate; λ∕4: quarter-wave plate[29]](/Images/icon/loading.gif)
Fig. 6. Schematic layout of resonant pumping Raman laser by direction control of field in the ring cavity. The Pump source is the SolsTis Ti:Sapphire laser from M Squared Lasers Ltd. HR: high reflector; PR: partial reflector; IC/OC: input/output coupler; DM: dichroic mirror, HR at Stokes, HT at pump; BS: uncoated beam sampler; BM: beam dump; λ ∕2: half-wave plate; λ ∕4: quarter-wave plate[29]
![[in Chinese]](/Images/icon/loading.gif)
Fig. 7. [in Chinese]
![(a) Temporal behavior of the forward Stokes and backward Stokes of the free-running Raman laser; (b) The Stokes trace of the free-running Raman laser by a scanning Fabry–Perot interferometer; (c) Temporal behavior of a unidirectional Raman laser using a feedback mirror. The inset shows stable single-mode operation of a scanning Fabry–Perot interferometer[29]](/Images/icon/loading.gif)
Fig. 7. (a) Temporal behavior of the forward Stokes and backward Stokes of the free-running Raman laser; (b) The Stokes trace of the free-running Raman laser by a scanning Fabry–Perot interferometer; (c) Temporal behavior of a unidirectional Raman laser using a feedback mirror. The inset shows stable single-mode operation of a scanning Fabry–Perot interferometer[29]
![Power stability of laser at highest output power[30]](/Images/icon/loading.gif)
Fig. 8. Power stability of laser at highest output power[30]
![Schematic layout of the experimental setup of the second Stokes operation in ring cavity by cavity locking. Inset is the coating curve of the M1 mirror, where spot P, S and SS means the reflectivity of the M1 for pump, 1st Stokes and 2nd Stokes fields[31]](/Images/icon/loading.gif)
Fig. 9. Schematic layout of the experimental setup of the second Stokes operation in ring cavity by cavity locking. Inset is the coating curve of the M1 mirror, where spot P, S and SS means the reflectivity of the M1 for pump, 1st Stokes and 2nd Stokes fields[31]
![Experimental setup of the single-longitudinal mode Raman lasers by self-mode supression effect of frequency doubling crystal[33]](/Images/icon/loading.gif)
Fig. 10. Experimental setup of the single-longitudinal mode Raman lasers by self-mode supression effect of frequency doubling crystal[33]
![Number of lasing modes of output power of 620 nm as a function of LBO temperature. The blue insets show spectra at temperatures of 35.0 °C and 39.2 °C[33]](/Images/icon/loading.gif)
Fig. 11. Number of lasing modes of output power of 620 nm as a function of LBO temperature. The blue insets show spectra at temperatures of 35.0 °C and 39.2 °C[33]
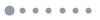
Fig. 12. (a) Frequency shift as a function of cavity length for output light; (b) Scanning Fabry–Perot interferometer trace of the single-frequency 589 nm laser
![Experimental setup of the single-longitudinal mode Raman lasers by using VBG[1]](/Images/icon/loading.gif)
Fig. 13. Experimental setup of the single-longitudinal mode Raman lasers by using VBG[1]
![(a) Stokes output spectra with and without optical feedback from the volume Bragg grating (VBG); (b) Temporal fluctuations of the center wavelength in 90 s measured at 0.5 W Stokes power[1]](/Images/icon/loading.gif)
Fig. 14. (a) Stokes output spectra with and without optical feedback from the volume Bragg grating (VBG); (b) Temporal fluctuations of the center wavelength in 90 s measured at 0.5 W Stokes power[1]
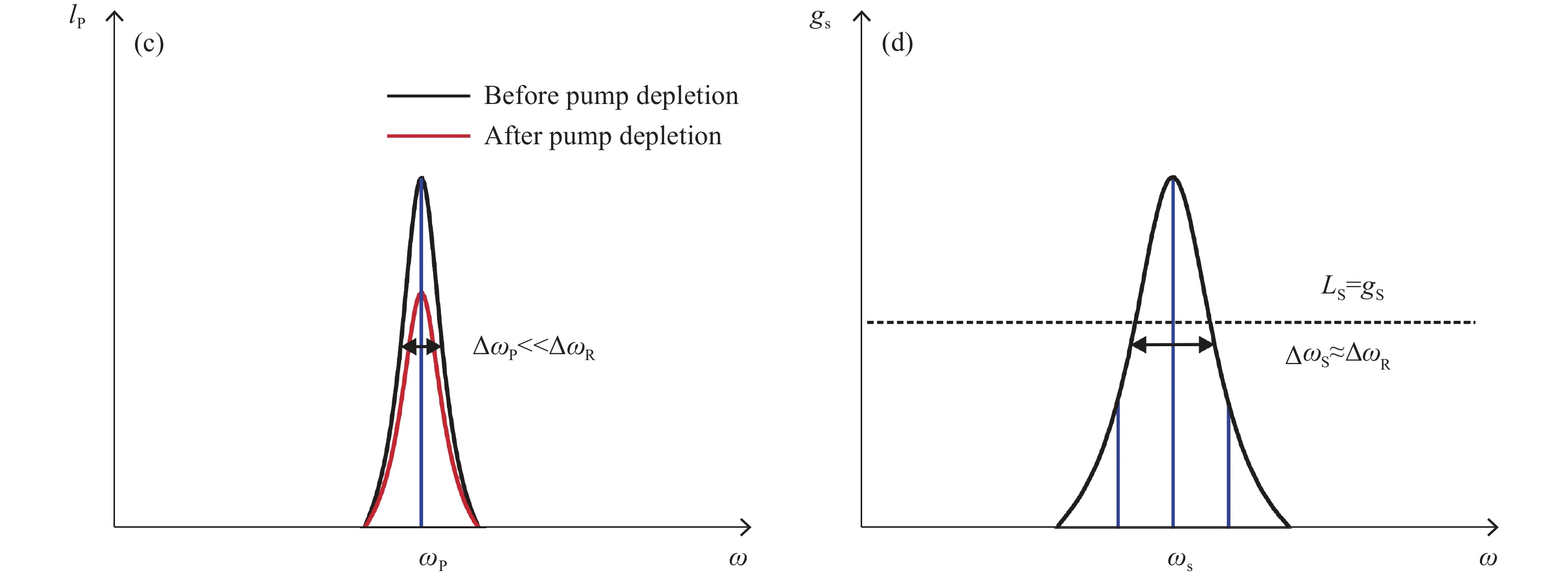
Set citation alerts for the article
Please enter your email address