Yue Li, Jian Li, Taixing Huang, Fei Huang, Jun Qin, Lei Bi, Jianliang Xie, Longjiang Deng, Bo Peng, "Active macroscale visible plasmonic nanorod self-assembled monolayer," Photonics Res. 6, 409 (2018)

Search by keywords or author
- Photonics Research
- Vol. 6, Issue 5, 409 (2018)
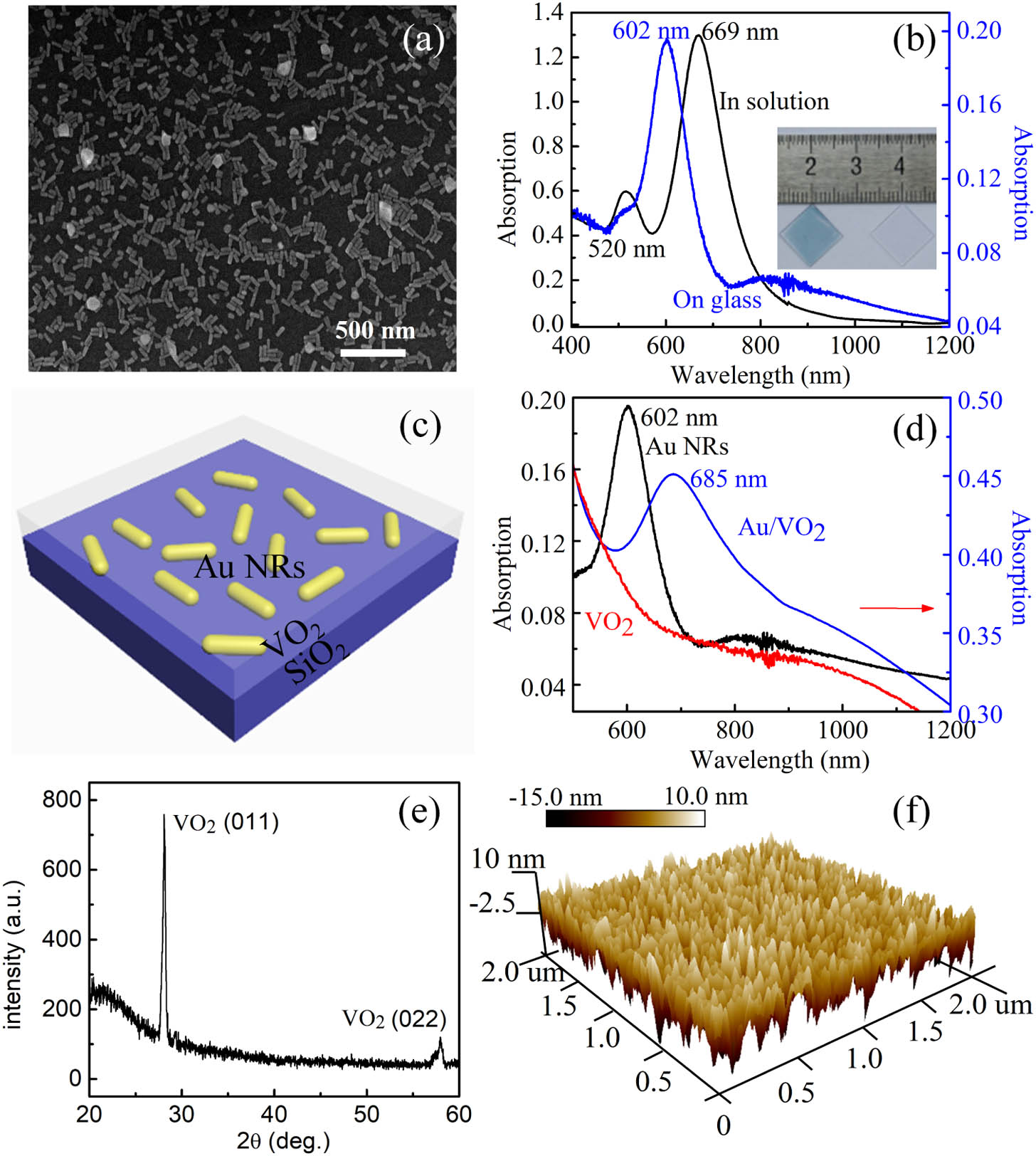
Fig. 1. (a) SEM image of Au NRs on quartz substrate. (b) Experimental absorption spectra of Au NRs dispersed in solution (black, left axis) and deposited on quartz substrate (blue, right axis). The inset is the photograph of Au NRs deposited on quartz substrate (left) and one blank quartz substrate (right). (c) Schematic of Au NRs on quartz substrate coated by VO 2 films. (d) The comparison of experimental absorption spectra for Au NRs (black), Au / VO 2 hybrid films (blue), and bare VO 2 films (red) on quartz substrate, respectively. (e) X-ray diffraction pattern and (f) AFM 3D image of VO 2 films deposited on quartz substrate.
![Experimental absorption spectra of bare VO2 and Au/VO2 films as a function of temperature. Heating [(a), (c)] and cooling [(b), (d)] on bare VO2 [(a), (b)] and Au/VO2 [(c), (d)] films. The arrows in (c) and (d) indicate the moving direction of the plasmonic peak with the change of temperature (red: heating; blue: cooling).](/richHtml/prj/2018/6/5/05000409/img_002.jpg)
Fig. 2. Experimental absorption spectra of bare VO 2 and Au / VO 2 films as a function of temperature. Heating [(a), (c)] and cooling [(b), (d)] on bare VO 2 [(a), (b)] and Au / VO 2 [(c), (d)] films. The arrows in (c) and (d) indicate the moving direction of the plasmonic peak with the change of temperature (red: heating; blue: cooling).
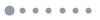
Fig. 3. (a), (b) Longitudinal plasmon resonance peak of Au / VO 2 films as a function of temperature. (c) Temperature hysteresis curves for the plasmon resonance peak of Au / VO 2 films. (d) Temperature hysteresis curves for the absorption variation (relative to the absorption intensity of 0.35) of bare VO 2 and Au / VO 2 films, taken at 685 nm.
![Raman spectra of bare VO2 [(a), (c)] and Au/VO2 [(b), (d)] films at different temperatures under optical pumping by 532 nm laser with the power of 0.2 mW [(a), (b)] and 0.5 mW [(c), (d)]. The black arrows represent the change of the temperature.](/Images/icon/loading.gif)
Fig. 4. Raman spectra of bare VO 2 [(a), (c)] and Au / VO 2 [(b), (d)] films at different temperatures under optical pumping by 532 nm laser with the power of 0.2 mW [(a), (b)] and 0.5 mW [(c), (d)]. The black arrows represent the change of the temperature.
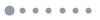
Fig. 5. Raman spectra of bare VO 2 and Au / VO 2 films at different power by 532 nm laser. (a)–(e) Comparison of Raman spectra between bare VO 2 (black) and Au / VO 2 (red) films at 0.2, 0.4, 0.5, 0.6, and 0.7 mW, respectively. (f) The intensity of 195 cm − 1 Raman peak in bare VO 2 and Au / VO 2 hybrid films as a function of laser power.
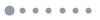
Fig. 6. Raman mapping of (a)–(c) bare VO 2 and (d)–(f) Au / VO 2 films under optical pumping by 532 nm laser at 0.6 mW. (a) and (d) 195 cm − 1 . (b) and (e) 223 cm − 1 . (c) and (f) 618 cm − 1 .
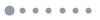
Fig. 7. White light reflection spectra for bare VO 2 and Au NRs / VO 2 films. (a), (b) Bare VO 2 films with the increase and decrease of temperature, respectively. (c) Bare VO 2 and (d) Au / VO 2 films excited by 633 nm laser at different laser powers. (e) Temperature hysteresis curves for the reflection intensity of bare VO 2 at 650 nm. (f) Comparison of reflection intensity at 650 nm in bare VO 2 (black, star) and Au / VO 2 (blue, dot) films as a function of laser power.
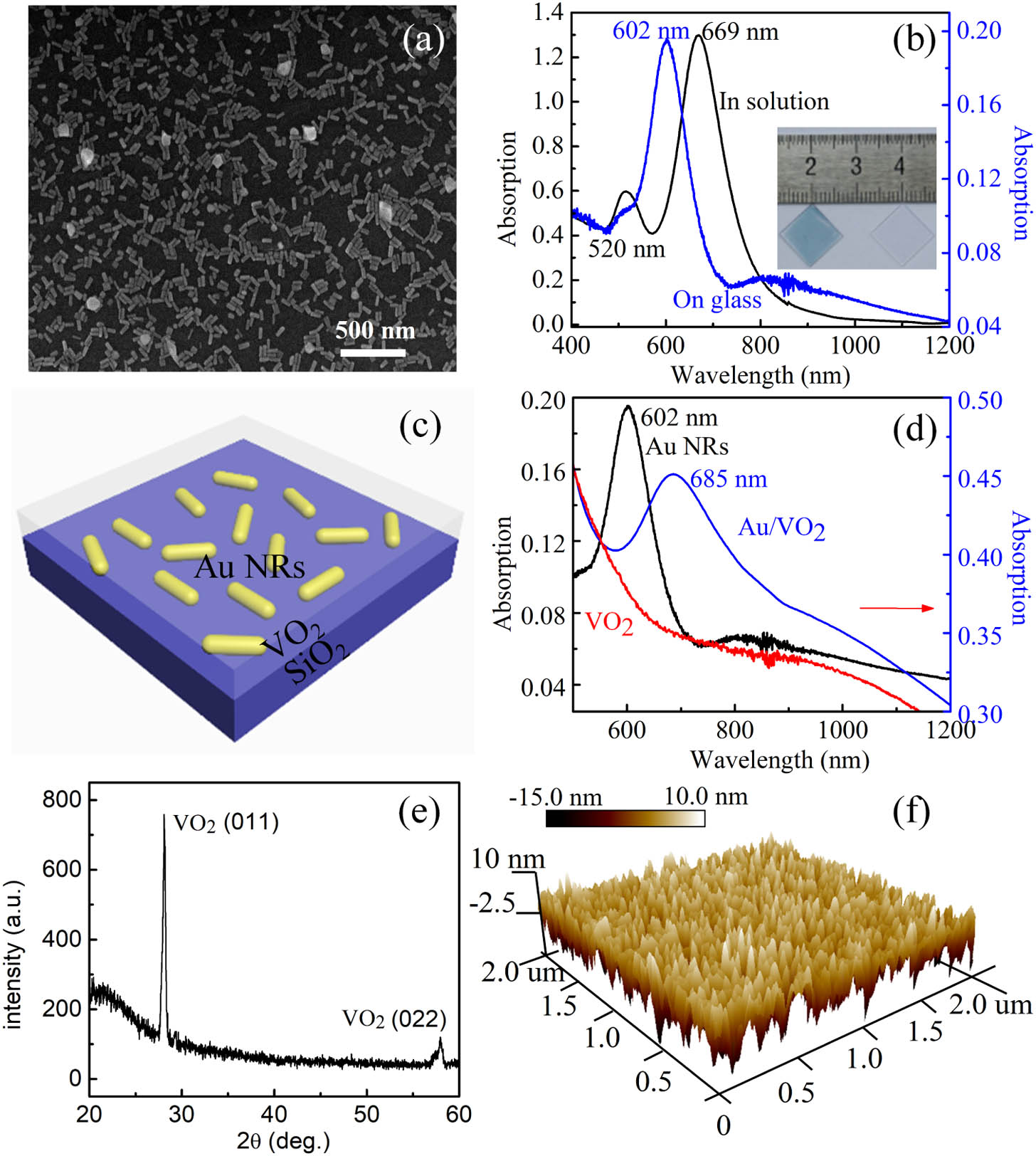
Set citation alerts for the article
Please enter your email address