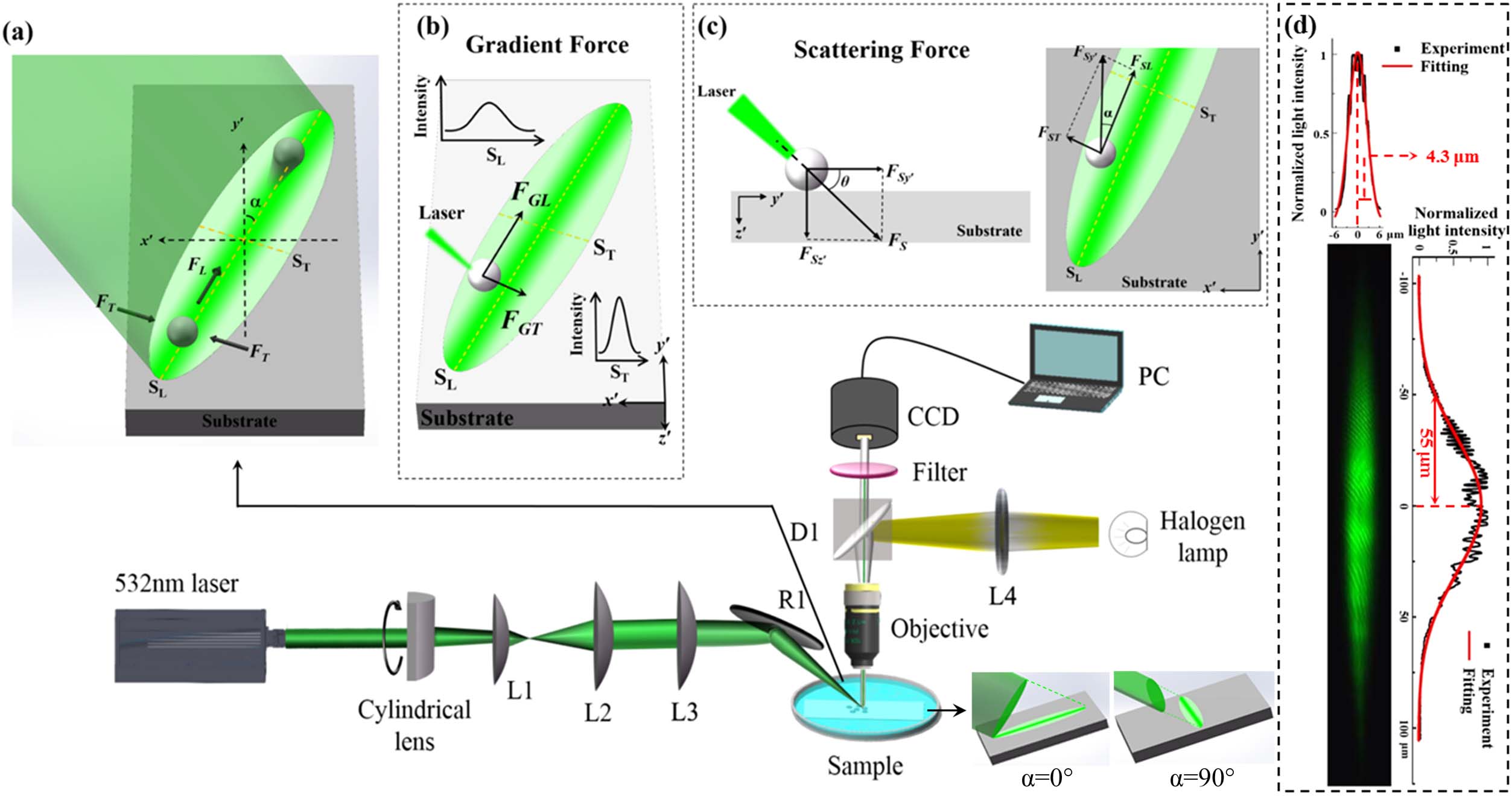
Fig. 1. Schematic diagrams of (a) the experimental setup and the opto-conveyor. R1, reflection mirror 1; L1, L2, L3, and L4, lenses. SL and ST denote the long and short axes of the opto-conveyor. FL and FT represent the driving and confining forces acting on the microparticle. The azimuthal angle α denotes the angle between the longitudinal direction and the y′ direction. The two insets show the schematic diagrams of the opto-conveyor for azimuthal angles of 0° and 90°, respectively. (b) The optical gradient force and (c) the optical scattering force (FS) acting on the microsphere, respectively. FGL and FGT denote the longitudinal and transverse components of the optical gradient forces, respectively. FSy′ and FSz′ denote the components of the optical scattering forces along the y′ and z′ directions. FST and FSL are the transverse and longitudinal components of FSy′, respectively. (d) The measured elliptical spot of the opto-conveyors on the substrate. The Gaussian waists along long and short axes are estimated as 55 μm and 4.3 μm, respectively.
Fig. 2. (a) Formation of the opto-conveyor on the substrate. The distributions of the electric intensity in (b) xoy, (c) xoz, and (d) yoz planes, respectively. The dimensionless optical force (DOF) of a microsphere with a radius r=3.5 μm when it moves along the (e) x, (f) y, and (g) z axes, respectively. Sx, Sy, and Sz are the relative positions of the microsphere along the x, y, and z axes.
Fig. 3. Confining forces (FT) for the azimuthal angles of (a) 0°, (b) 45°, and (c) 90°, respectively. The red dotted lines show the equilibrium points of the confining force. Driving forces (FL) for the azimuthal angles of (d) 0°, (e) 45°, and (f) 90°, respectively. The black dotted lines show the positions of the maximum driving force for the azimuthal angles of 0° and 45°, and the equilibrium point of the positive and negative driving force for the azimuthal angle of 90°, respectively. ST and SL are the relative positions of the microsphere along the short and long axes of the opto-conveyor, respectively.
Fig. 4. (a) Targeting delivery processes and (b) the delivery trajectories of the PS microparticles at six different start points. The yellow dotted lines show the trajectories of microparticle movement. The green ellipses denote the opto-conveyor, and the reddish dots denote the positions of the microparticles every second.
Fig. 5. Delivery processes of the microspheres for five different optical powers of 221.7 mW, 337.6 mW, 484.1 mW, 702.5 mW, and 769.4 mW, respectively. The incident angle θ is 30°, and the azimuthal angle α is 0°. The yellow dotted lines show the trajectories of microparticle movement, and the reddish dots denote the positions of the microparticles every second.
Fig. 6. Speed distributions during microparticle delivery processes as functions of (a) time and (b) position for five different optical powers of 221.7 mW, 337.6 mW, 484.1 mW, 702.5 mW, and 769.4 mW in the experiments, respectively. (c) The theoretical and experimental distributions of the transport speed and driving force at the optical power of 337.6 mW. (d) The experimental and theoretical maximum speed and the delivery time consumption versus the optical power, respectively.
Fig. 7. (a) Relationship between the irradiation time, optical power, and the delivery distance, and (b) relationship between the irradiation time and the delivery distance at the optical power of 484.1 mW. The dotted lines show the delivery distance at the irradiation times of 4 s, 5 s, 6 s, and 22 s, respectively.
Fig. 8. Delivery distances of microparticles at the optical power of 484.1 mW with the delivery periods of 4 s, 5 s, 6 s, and 26 s, respectively. The incident angle θ is 30°, and the azimuthal angle α is 0°.
Fig. 9. (a) Delivery trajectories for different azimuthal angles at the optical power of 484.1 mW and the incident angle of 45°. The optical images of the opto-conveyors are shown in each inset. (b) The experimental and theoretical delivery distances, and (c) the experimental and theoretical maximum speeds of the microparticles versus azimuthal angles.
Fig. 10. Simultaneous delivery of microparticles to a targeting object at the optical power of 484.1 mW, the incident angle of 30°, and the azimuthal angle of 0°. The dotted lines denote the opto-conveyor.
Fig. 11. Geometry for calculating the optical force due to the scattering of incident rays by a microsphere.
| Delivery Distance | Period (s) | Theory (μm) | Experiment (μm) | Deviation (%) | 4 | 58 | 58 | 0 | 5 | 136 | 134 | 1.47 | 6 | 158 | 152 | 3.80 | 22 | 210 | 211 | 0.48 |
|
Table 1. Theoretical and Experimental Results on the Control of the Delivery Distance