Author Affiliations
1State Key Laboratory of Fabrication Technologies for Integrated Circuits, Institute of Microelectronics, Chinese Academy of Sciences, Beijing 100029, China2Frontier institute of Chip and System, Fudan University, Shanghai 200433, China3College of Electronic Science and Technology, National University of Defense Technology, Changsha 410073, China4University of Chinese Academy of Sciences, Beijing 100049, Chinashow less
Abstract
The performance and reliability of ferroelectric thin films at temperatures around a few Kelvin are critical for their application in cryo-electronics. In this work, TiN/Hf0.5Zr0.5O2/TiN capacitors that are free from the wake-up effect are investigated systematically from room temperature (300 K) to cryogenic temperature (30 K). We observe a consistent decrease in permittivity (εr) and a progressive increase in coercive electric field (Ec) as temperatures decrease. Our investigation reveals exceptional stability in the double remnant polarization (2Pr) of our ferroelectric thin films across a wide temperature range. Specifically, at 30 K, a 2Pr of 36 μC/cm2 under an applied electric field of 3.0 MV/cm is achieved. Moreover, we observed a reduced fatigue effect at 30 K in comparison to 300 K. The stable ferroelectric properties and endurance characteristics demonstrate the feasibility of utilizing HfO2 based ferroelectric thin films for cryo-electronics applications.Introduction
HfO2 based ferroelectric thin films have attracted wide interest since their first discovery in 2011[1] due to their encouraging properties, including complementary metal−oxide−semiconductor (CMOS) compatibility, physical thickness scalability[2], low power consumption and fast speed[3], the HfO2 based ferroelectric devices have demonstrated potential for broad applications including non-volatile memory[4] and bio-inspired computing[5]. Among various doped and undoped HfO2 based ferroelectric thin films, the hafnia-zirconia solid solution (HZO) films show robust ferroelectricity within a wide composition range, and their relatively low annealing temperature (350−500 °C) makes them potentially compatible with the Back-End-of-Line (BEOL) process.
Nowadays, the rapid development of space and quantum computing applications calls for high-performance memory devices that can reliably operate at cryogenic temperatures (below about 120 K)[6]. While the research on this subject is currently limited, previous works have proved that memory devices with HfO2 based ferroelectric materials or amorphous dielectrics showing ferroelectric-like electrical behaviors can be one of the most promising candidates for cryo-electronics[7−15]. Müller et al. investigated the temperature-dependent polarization-electric field (P−E) hysteresis in Hf0.3Zr0.7O2 thin films and observed a transition from anti-ferroelectric-like to ferroelectric behavior with the temperature decreasing from 230 to 80 K[7]. Henry et al. first demonstrated ferroelectric switching in Hf0.58Zr0.42O2 thin films with NbN electrodes at 4 K[8]. Moreover, Hur et al. thoroughly characterized the electrical performance of Hf0.5Zr0.5O2 ferroelectric capacitors with TiN electrodes from 400 to 4 K[9]. However, the initial findings were obtained using non-optimized HZO devices, which exhibited either anti- or weak-ferroelectric behavior in their pristine states, and required a wake-up operation using bipolar voltage cycling to achieve the commonly reported ferroelectric full-hysteresis loops. The wake-up process is not practical for real products and has been widely attributed to the redistribution of defects and/or the phase transformation from the non-ferroelectric phase to the ferroelectric one in previous literature[16]. Efficient ways to mitigate the wake-up issue have been extensively explored and demonstrated, including defects and interfacial engineering, plasma treatment, and epitaxial growth[17]. It has been observed that the wake-up process can be effectively suppressed under low temperatures[9]. Consequently, to unlock the full potential of these materials, it is imperative to investigate the temperature dependence on the ferroelectric properties of wake-up free ferroelectric HZO capacitors (referred to as optimized HZO capacitors) down to cryogenic temperatures.
In this letter, we present systematic electrical characterizations on wake-up free TiN/HZO/TiN ferroelectric capacitors, showcasing their stable ferroelectric performance over a wide temperature range from 300 down to 30 K. Our work demonstrates the great potential of HZO ferroelectric capacitors for cryogenic electronics applications.
Experiments
The TiN/Hf0.5Zr0.5O2/TiN capacitor devices were fabricated on SiO2/Si substrates. A blanket layer of 40 nm TiN as bottom electrodes (BEs) was deposited by sputtering. The 10-nm-thick HZO thin films were then prepared by thermal atomic layer deposition with a substrate temperature of 280 °C. [(CH3)(C2H5)N]4Hf (TEMAH), [(CH3)(C2H5)N]4Zr (TEMAZ) and H2O were used as Hf precursor, Zr precursor and oxygen source, respectively. The Hf : Zr ratio of 1 : 1 is controlled by alternating HfO2 and ZrO2 deposition cycles. The 40 nm sputtered TiN top electrodes (TEs) with an area of 50 × 50 μm2 were patterned by the standard photolithography and lift-off process. Finally, the fabricated capacitors were annealed at 500 °C for 30 s by rapid thermal annealing in nitrogen atmosphere.
The crystal structure of HZO thin films after TEs removal was analyzed by grazing incidence X-ray diffraction (GI-XRD) at an incident angle of 0.5°, employing Bruker D8 Advance equipment. The transmission electron microscope (TEM) image was acquired using the FEI 200 kV Titan Themis STEM equipment. The temperature-dependent electrical characterizations were performed in a Lake Shore cryogenic probe station. The capacitance−electric field (C−E) and leakage−electric field (J−E) characteristics were measured by a Keithley 4200 semiconductor parameter analyzer, while the polarization-electric field (P−E) hysteresis loops, positive-up-negative-down (PUND) behaviors and endurance were characterized by a Precision LC Ⅱ ferroelectric tester (radiant technologies). The direct-current J−E curves were collected during the voltage swept from 3 to 0 V and −3 to 0 V. As schematically shown in the inset of Fig. 1 (a), TEs were biased with BEs grounded during the electrical measurements.
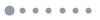
Figure 1.(Color online) (a) The P−E hysteresis curves of HZO capacitor at its pristine state and after 103 cycles. The inside illustration is a schematic diagram of HZO capacitor devices during electrical measurements. (b) The GI-XRD diffractogram of HZO thin film at an incident angle of 0.5°. (c) A cross-sectional TEM image of the HZO capacitor. (d) The fast Fourier transform pattern from the HR-TEM image in the yellow box in (c).
Results and discussion
Fig. 1(a) shows the P−E hysteresis curves of our optimized HZO capacitor at its pristine state (blue curve) and after 103 cycles (red curve), confirming its wake-up free behavior. The crystal structure of our HZO thin film was analyzed by GI-XRD (Fig. 1(b)), where diffraction peaks that possibly correspond to (111) and (200) reflections of the orthorhombic (o-) phase can be identified. The cross-sectional TEM image of the HZO capacitor is shown in Fig. 1(c), which illustrates that the thickness of the HZO film is 10 nm, and the interfaces between HZO and electrodes are clean and uniform. The fast Fourier transform pattern of the selected region (yellow dash box in Fig. 1(c)) demonstrates the existence of a ferroelectric o-phase (space group: Pbc21) in our HZO thin film (Fig. 1 (d)).
To examine the leakage and its temperature dependence of our HZO capacitors, the direct-current J−E characteristics at various temperatures are shown in Fig. 2 (a). The low leakage current density of 4.8 A/cm2 (leakage current of 1.2 nA) at 300 K and 2 MV/cm indicates a good insulating quality of the HZO thin films. The leakage decreases with the decrease in temperature. Figs. 2 (b) and 2(c) show the fitting results of the leakage current at temperatures from 300 K to 130 K by the Poole−Frenkel (P−F) emission and at 30 K by the Fowler−Nordheim (F−N) tunneling, respectively. The P−F emission, where the current density is directly dependent on the temperature, dominates the leakage in the HZO capacitor when the temperature decreases from 300 K[18], which explains the observed decrease in leakage with temperature, while the F−N tunneling becomes dominant when the temperature is lower than 77 K. Fig. 2(d) shows the P−E hysteresis loops measured under 3.0 MV/cm from room temperature to 30 K. To accurately determine switching polarization while mitigating non-switching interference, the PUND scheme is employed (inset in Fig. 2(e)). The 2Pr of our HZO ferroelectric capacitors remains almost unchanged 36 µC/cm2 at 30 K with an increase in the coercive electric field (Ec) as the temperature decreases, which will be discussed further in the following paragraph. Fig. 2(e) shows the statistical results of ±Pr values at varied temperatures from 10 randomly picked devices, demonstrating the great uniformity of the HZO capacitors, which is consistent with the studies by Jiang et al. across a 12-inch wafer[19]. As expected, permittivity- electric field (εr−E) measurements (Fig. 2(f)) of the HZO capacitors show typical butterfly shaped hysteresis across the whole temperature range. The εr−E curves were measured under 2 MV/cm to avoid the device breakdown during measurements. The capacitance peaks in the εr−E curves that correlate with +Ec and −Ec, shifted to the right-hand and left-hand, respectively, which aligns with the trend observed in P−E measurements. In agreement with previous observations[13], the permittivity of our capacitor (@ 2 MV/cm) monotonically decreases from 31.1 to 28.1 when the temperature decreases from 300 to 30 K.
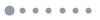
Figure 2.(Color online) (a) The J−E curves of TiN/HZO/TiN capacitor ranging from 300 to 30 K. (b) The fitting results of the leakage current at temperatures from 300 to 130 K by the P−F emission at 300, 200, and 130 K. (c) The fitting plot of the leakage current at 30 K by the F−N tunneling. (d) The typical P−E curves of HZO capacitors at temperatures from 300 to 30 K under 3.0 MV/cm. (e) The statistical results of ±Pr values at temperatures from 300 to 30 K. The inset shows the typical PUND curves at various temperatures under 3.0 MV/cm. (f) εr−E curves of HZO capacitors in the temperature ranging from 300 to 30 K.
To draw more solid conclusions on the temperature dependence of 2Pr and the averaged Ec (defined as (|+Ec| + |−Ec|)/2), we performed PUND measurements on our HZO ferroelectric capacitors under different electric fields. Figs. 3(a)−3(c) show the PUND hysteresis loops measured from 300 to 30 K under 2.5, 2.0, and 1.5 MV/cm, respectively. These show that the temperature dependence of ferroelectric properties is strongly influenced by the applied electric field[11]. As shown in Fig. 3(d), the averaged Ec monotonically increases as the temperature decreases, suggesting a higher barrier for ferroelectric switching at lower temperatures[20]. In particular, under 3.0 MV/cm, the averaged Ec increases from 1.1 MV/cm (@ 300 K) to 1.4 MV/cm (@ 30 K) as the temperature decreases. It should be noted that here we have defined the electric field when the polarization becomes zero as ±Ec for all measurements with different temperatures and maximum E-fields. For cases that the polarization cannot be fully switched, e.g. at 1.5 MV/cm and 30 K, ±EP=0 can be a more applicable alternative for the definition above. In Fig. 3(e), the HZO capacitor shows constant 2Pr of ~36 µC/cm2 under 3.0 MV/cm and ~30 µC/cm2 under 2.5 MV/cm down to 30 K. Meanwhile, with an applied electric field amplitude (1.5 MV/cm) comparable to coercive electric field, the decrease in temperatures leads to a clear reduction in 2Pr (from 12.5 µC/cm2 at 300 K to 2.9 µC/cm2 at 30 K). Fig. 3(f) summarizes the temperature-dependent 2Pr of HZO ferroelectric capacitors from previous literatures and our work. Our devices exhibited negligible changes and achieved the highest 2Pr at cryogenic temperatures, which can be attributed to the high quality of the ferroelectric HZO thin film and the well-optimized oxide/electrode interfaces, highlighting the benefit of their nature of wake-up free.
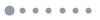
Figure 3.(Color online) The PUND curves of HZO capacitors at different temperatures under (a) 2.5 MV/cm, (b) 2.0 MV/cm, and (c) 1.5 MV/cm. (d) The averaged Ec values at different temperatures. (e) The 2Pr values at different temperatures. (f) The relationship between 2Pr values with temperature in previous reports and this work. The 2Pr values in this work are from PUND data under 3.0 and 2.5 MV/cm. The letter W and P stand for woken-up state and pristine state, respectively. The applied electric fields are indicated on the right.
Finally, the endurance of our TiN/HZO/TiN capacitors at 300 and 30 K is characterized and compared, as shown in Fig. 4. For the endurance measurements, repeated pulses (electric field of 2.5 MV/cm and pulse width of 1 µs) are applied. At room temperature, the 2Pr of the HZO capacitor dropped significantly from 36.4 µC/cm2 at its pristine state to 22.4 µC/cm2 after 3 × 106 cycles. While at 30 K, there was only a slight reduction in the 2Pr value from 36.9 to 31.5 µC/cm2 after 3 × 106 cycles, similar to the observation by Hsiang et al[21]. The fatigue in HfO2 based ferroelectrics has been widely attributed to the generation and distribution of charged defects, e.g. oxygen vacancies[22]. These two processes can be suppressed under low temperatures, which gives rise to mitigated fatigue effect and enhances the suitability of HZO ferroelectric capacitors for cytogenetic applications.
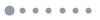
Figure 4.(Color online) Endurance characteristics of HZO capacitors under 2.5 MV/cm at 300 and 30 K.
Conclusion
In summary, our study comprehensively investigates the ferroelectric characterization of wake-up free TiN/HZO/TiN capacitors, including J−E, P−E, εr−E, and endurance measurements, spanning a wide temperature range from 300 to 30 K. Our findings highlight the promising ferroelectric and endurance properties of HZO films at cryogenic temperatures. Notably, the 2Pr values of HZO thin films remain mostly stable down to 30 K. Moreover, the HZO capacitors show weaker fatigue characteristics at 30 K compared to room temperature, indicating their potential for cryogenic applications. Precise computational analysis and microscopic characterizations under different temperatures are further required to establish the relationship between electrical properties and structural evolution to better understand and advance the application of these materials in various technological fields.
References
[1] T S Böscke, J Müller, D Bräuhaus et al. Ferroelectricity in hafnium oxide thin films. Appl Phys Lett, 99, 102903(2011).
[2] S S Cheema, D Kwon, N Shanker et al. Enhanced ferroelectricity in ultrathin films grown directly on silicon. Nature, 580, 478(2020).
[3] W Banerjee, A Kashir, S Kamba. Hafnium oxide (HfO2)–A multifunctional oxide: A review on the prospect and challenges of hafnium oxide in resistive switching and ferroelectric memories. Small, 18, 2107575(2022).
[4] A I Khan, A Keshavarzi, S Datta. The future of ferroelectric field-effect transistor technology. Nat Electron, 3, 588(2020).
[5] R R Cao, X M Zhang, S Liu et al. Compact artificial neuron based on anti-ferroelectric transistor. Nat Commun, 13, 7018(2022).
[7] J Müller, T S Böscke, U Schröder et al. Ferroelectricity in simple binary ZrO2 and HfO2. Nano Lett, 12, 4318(2012).
[8] M D Henry, S W Smith, R M Lewis et al. Stabilization of ferroelectric phase of Hf0.58Zr0.42O2 on NbN at 4 K. Appl Phys Lett, 114, 092903(2019).
[9] J Hur, Y C Luo, Z Wang et al. Characterizing ferroelectric properties of Hf0.5Zr0.5O2 from deep-cryogenic temperature (4 K) to 400 K. IEEE J Explor Solid State Comput Devices Circuits, 7, 168(2021).
[10] M H Park, C C Chung, T Schenk et al. Origin of temperature-dependent ferroelectricity in Si-doped HfO2. Adv Elect Materials, 4, 1700489(2018).
[11] D Y Zhou, Y Guan, M M Vopson et al. Electric field and temperature scaling of polarization reversal in silicon doped hafnium oxide ferroelectric thin films. Acta Mater, 99, 240(2015).
[12] D Wang, J L Wang, Q A Li et al. Stable ferroelectric properties of Hf0.5Zr0.5O2 thin films within a broad working temperature range. Jpn J Appl Phys, 58, 090910(2019).
[13] M Pešić, F P G Fengler, L Larcher et al. Physical mechanisms behind the field-cycling behavior of HfO2-based ferroelectric capacitors. Adv Funct Materials, 26, 4601(2016).
[14] J W Adkins, I Fina, F Sánchez et al. Thermal evolution of ferroelectric behavior in epitaxial Hf0.5Zr0.5O2. Appl Phys Lett, 117, 142902(2020).
[15] H Liu, Q Y Yang, C J Jin et al. Mobile-ionic FETs with ultra-scaled amorphous dielectric achieving ferroelectric behaviors and sub-kT/q swing with temperature down to 77 K. Sci China Inf Sci(2023).
[16] P F Jiang, Q Luo, X X Xu et al. Wake-up effect in HfO2-based ferroelectric films. Adv Elect Materials, 7, 2000728(2021).
[17] J P B Silva, K C Sekhar, R F Negrea et al. Progress and perspective on different strategies to achieve wake-up-free ferroelectric hafnia and zirconia-based thin films. Appl Mater Today, 26, 101394(2022).
[18] M H Park, H J Kim, Y J Kim et al. Study on the internal field and conduction mechanism of atomic layer deposited ferroelectric Hf0.5Zr0.5O2 thin films. J Mater Chem C, 3, 6291(2015).
[19] H Jiang, M A Bhuiyan, Z Liu et al. A study of BEOL processed Hf0.5Zr0.5O2- based ferroelectric capacitors and their potential for automotive applications(2020).
[20] M H Park, H J Kim, Y J Kim et al. Giant negative electrocaloric effects of Hf0.5Zr0.5O2 thin films. Adv Mater, 28, 7956(2016).
[21] K Y Hsiang, J Y Lee, Z F Lou et al. Cryogenic endurance of anti-ferroelectric and ferroelectric Hf1-xZrxO2 for quantum computing applications(2023).
[22] J Chen, C Jin, X Yu et al. Impact of oxygen vacancy on ferroelectric characteristics and its implication for wake-up and fatigue of HfO2-based thin films. IEEE Trans Electron Devices, 69, 5297(2022).