David Allioux, Ali Belarouci, Darren Hudson, Eric Magi, Milan Sinobad, Guillaume Beaudin, Adrien Michon, Neetesh Singh, Regis Orobtchouk, Christian Grillet, "Toward mid-infrared nonlinear optics applications of silicon carbide microdisks engineered by lateral under-etching [Invited]," Photonics Res. 6, B74 (2018)

Search by keywords or author
- Photonics Research
- Vol. 6, Issue 5, B74 (2018)
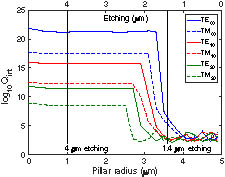
Fig. 1. Evolution of the theoretical intrinsic Q factor with the radius of the pillar at λ = 1.55 μm for a 10 μm disk with perfect edges. The six radial-order modes with the highest theoretical Q factors and lowest effective areas are plotted. The single-mode operation for the TE mode is achieved with a pillar radius around 3.5 μm.
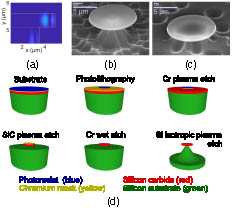
Fig. 2. (a) Intensity mode profile of a simulated WGM at λ = 1.55 μm in a 10 μm disk with 4 μm lateral under-etching (FVFD mode solver). The mode is a second-order TE mode leaking in the Si. Scanning electron microscope images of 10 μm disks with (b) 4 μm and (c) 1.4 μm lateral under-etching, and (d) fabrication process.
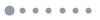
Fig. 3. (a) Scheme of the evanescent coupling technique, and (b) coupling between the tapered fiber and a 10 μm disk.
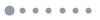
Fig. 4. (a) Experimental transmitted spectrum for a 10 μm diameter disk with 4 μm of lateral under-etching. Two polarizations are plotted: 0° in blue and 90° in red. Note that some modes appear in both polarizations. Below, two FSRs are plotted. Using mode solver, we inferred that they correspond to the TE 20 modes for the dark one and to the TE 40 for the green one. The power mode profiles are represented in (b) and (c), respectively.
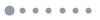
Fig. 5. (a) Experimental (blue) and simulated (red) TM normalized transmitted spectra for a 10 μm diameter disk with 4 μm Si lateral under-etching. (b) TM (blue) and TE (black) polarizations of the transmitted spectra for a 10 μm diameter disk with a 4 μm lateral under-etching.
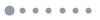
Fig. 6. Effective index as a function of wavelength of the WGMs for a 10 μm disk with (a) 4 μm and (b) 1.4 μm Si lateral under-etching.
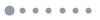
Fig. 7. (a) Experimental transmission spectrum from a 10 μm diameter disk with 1.4 μm Si lateral under-etching in blue. Equivalent simulated transmission spectrum with FDTD in red and mode solver resonances in green. (b) Zoom of the resonance. (c) Power mode profile obtained with mode solver.
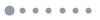
Fig. 8. Group velocity dispersion D λ evolution with radius (x axis) and height (y axis) for a SiC microdisk at λ = 4 μm wavelength. The dotted red line indicates the zero of dispersion. The dashed gray lines indicate the effective area of the mode in square micrometers.
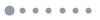
Fig. 9. Dispersion parameter D λ evolution with radius (x axis) and wavelength (y axis) for a SiC microdisk with a height of 800 nm. The dotted red line indicates the zero of dispersion. The dashed gray lines indicate the effective area of the mode in square micrometers.
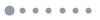
Fig. 10. Theoretical maximum generated frequency comb bandwidth as a function of FSR for a pump power of 1.5 W, a GVD D λ = 1 ps / ( nm · km ) , and a wavelength of 4 μm as obtained with Eq. (1 ). The different curves are for Q factors from 1 × 10 4 to 5 × 10 6 . The dotted line indicates the bandwidth needed to achieve an octave spanning from 2.7 to 5.4 μm.
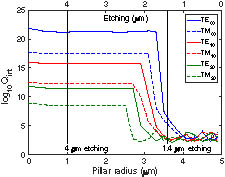
Set citation alerts for the article
Please enter your email address