Author Affiliations
1Light, nanomaterials, nanotechnologies (L2n), ERL 7004, CNRS. University of Technology of Troyes, 12 rue Marie Curie, 10004 Troyes Cedex, France2Teem Photonics, 61 Chemin du Vieux Chêne, 38246 Meylan, France3Key Laboratory of Advanced Display and System Application, Ministry of Education, School of Mechatronic Engineering and Automation, Shanghai University, Shanghai 200072, China4Sino-European School of Technology, Shanghai University, Shanghai 200044, China5e-mail: christophe.couteau@utt.fr6e-mail: sylvain.blaize@utt.frshow less
Fig. 1. (a) Schematic of the conventional DLW-TPP platform. (b), (c) Cross section and 3D illustration of our developed DLW-TPP platform for thick substrates. The red dots represent QDs dispersed inside the polymer liquid. The white dashed line shows the control of the laser focus height, which is indicated with H. (d) Schematic of a single QDs-polymer voxel presenting a typical oval shape.
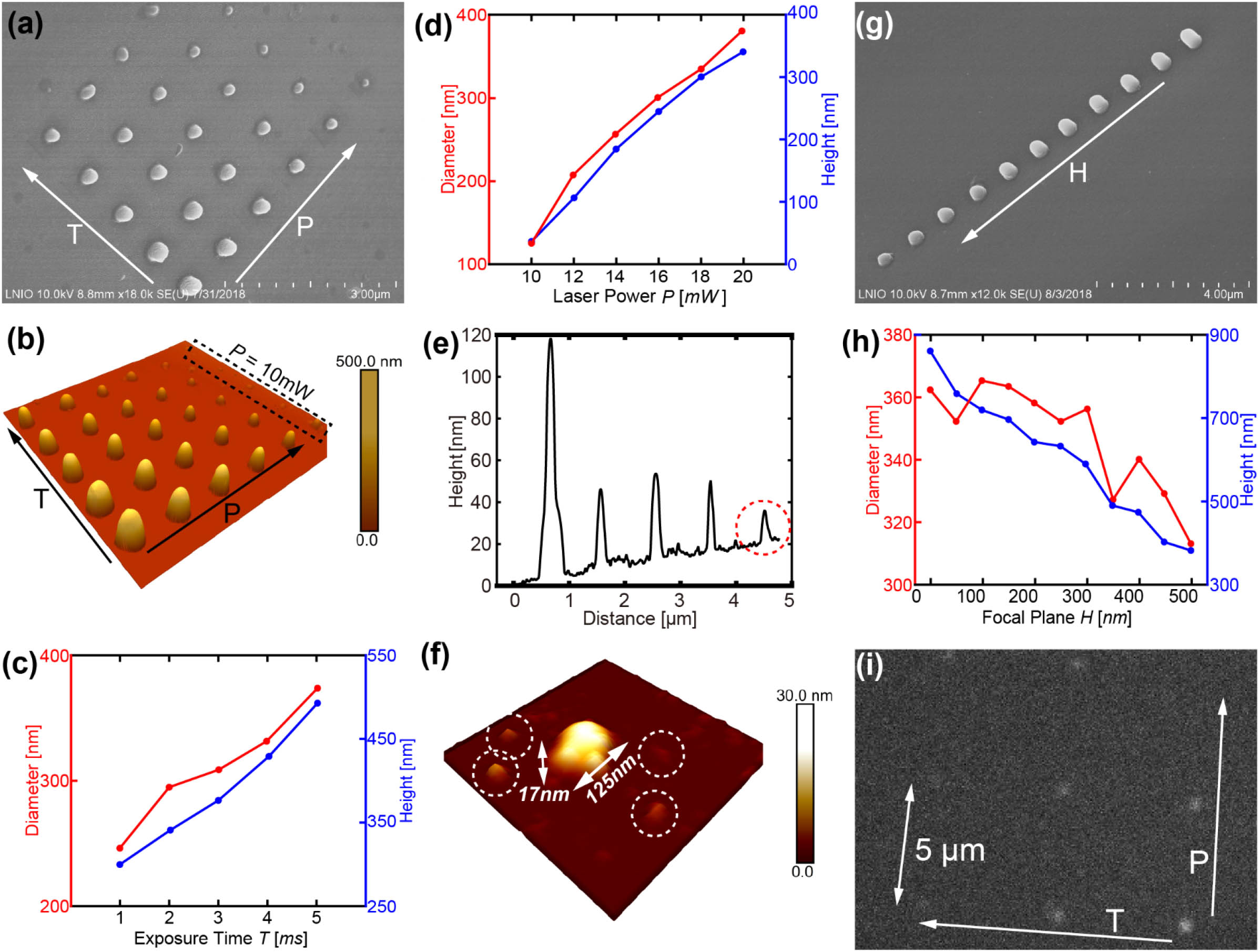
Fig. 2. Characterization of QD-polymer voxels. Tilt-view (a) SEM image and (b) AFM image of the voxel array with laser powers P (10 to 20 mW using 2 mW steps) and exposure time T (1 to 5 ms using 1 ms steps). The default value H in this sample is 0 nm. (c), (d) Dependence of the height and diameter of single voxel structures in function of P and T, respectively. P=20 mW in (c), T=5 ms in (d). (e) AFM line profile of the QD-polymer voxels with P=10 mW marked with black dashed area in (b). The peak marked with the red dashed circle indicates the profile of the smallest voxel with T=1 ms, which is shown in the AFM image (not to scale) in (f). Four dots marked with white dashed circles are QDs surrounded by a polymer layer. Tilt-view (b) SEM image and (h) size dependence of an array of voxel structures by only changing the focal plane H (0 to 500 nm using 50 nm steps), with P=20 mW and T=5 ms. (i) Measured PL image of a voxel array with 5 μm of distance between voxels.
Fig. 3. (a) SEM image of IEWs coated with a 4 nm thick conducting carbon layer. The schematic 3D view of the IEWs is shown in the inset. Integration of single QD-polymer voxels (b) on top and at the center of different waveguides and (c) several on the same waveguide, respectively. (d) SEM image of a single QD-polymer voxel on a single IEW; the inset shows the enlarged SEM image of the structure. (e) Far-field emission of the voxel in (d). The white dashed line indicates the outline of the IEW.
Fig. 4. (a) Schematic of QD emission measurement. (b) Spectrum of far-field emission emitted from the single QD-polymer nanostructure on the IEW with a Gaussian fit (red line). (c) Normalized extracted spectrum collected from the waveguide facet by a single mode fiber. The black line is for the nanoemitters placed on the IEW, while the gray line is for the IEW without nanoemitters on top.
Fig. 5. (a)–(d) Fabrication of a single layer of QDs by controlling laser printing parameters (P,T,H). (e) SEM image of a single QD layer of the QD-polymer nanocomposite; this sample is the same as (d). The inset shows the detailed part marked with a yellow square.
Fig. 6. Relationship between QD concentration and far-field PL of QD-polymer voxels.
Fig. 7. (a) Refractive index distribution of the IEW. (b) |E| field cross-section distribution with a 590 nm TE propagating mode along the IEW; the white solid line indicates the amplitude distribution vertical to the interface.
Fig. 8. (a) Experimental fiber-IEW coupling stage. (b) Fiber coupled with the IEWs sample. (c) Microcopy image of fiber-IEWs coupling, enlarging the yellow dashed square in (b). (d) Coupled alignment laser scattered by QD-polymer voxel. White dashed line represents the corresponding waveguide.
Fig. 9. QD-IEW coupling efficiency as a function of the QD position along (a) x and (b) z.
Fig. 10. (a) Schematic of an ensemble PL spectrum that consists of the individual QD emission spectrum convolved with the interparticle inhomogeneities. (b) Simulated transmission spectrum for 11 QDs emission coupled into IEW propagating modes.