
- Chinese Optics Letters
- Vol. 20, Issue 2, 021402 (2022)
Abstract
1. Introduction
Fiber lasers have already found a wide variety of applications ranging from scientific research and medical treatment to industrial processing[
In fact, there are several approaches to realizing mode adjusting/switching in fiber lasers[
In this Letter, we demonstrate a hundred-watt-level spatial mode switchable fiber laser system adopting an AIFG. The output beam profiles can be switched from the
Sign up for Chinese Optics Letters TOC. Get the latest issue of Chinese Optics Letters delivered right to you!Sign up now
2. Experimental Setup
The experiment system is based on a master oscillator power amplifier (MOPA) structure, which consists of a mode switchable seed fiber laser operating at
Figure 1.(a) Experimental setup of the mode switchable fiber laser based on a master oscillator power amplifier scheme; (b) schematic of the intra-cavity mode switchable seed fiber laser. AIFG, acoustically induced fiber grating; RF, radio frequency; LD, laser diode; PSC, pump and signal combiner; YDF, ytterbium-doped fiber; CLS, cladding light stripper; QBH, quartz block holder; FLM, fiber loop mirror; MFA, mode field adapter; PC, polarization controller; FBG, fiber Bragg grating.
The few-mode FBG and the AIFG are first characterized with the methods illustrated in our previous work[
Figure 2.(a) Transmission and reflection spectra of the few-mode FBG used in the intra-cavity mode switchable fiber oscillator; (b) transmission spectra of the AIFG under different modulation frequencies.
3. Results and Discussion
As for the MOPA shown in Fig. 1(a), the output mode from the 1070 nm seed laser could be manipulated by actively controlling the applied RF signal on the AIFG. The output mode would be switched from the
Figure 3.(a) Output power of the LP01 and LP11 modes from the fiber amplifier as a function of the pump power (inset: beam profiles of the LP11 mode); (b) output spectra of the LP01 and LP11 modes under the maximum output power (inset: output spectra of the seed laser).
Furthermore, as shown in Fig. 1(b), an intra-cavity mode switchable seed fiber oscillator is built by integrating an AIFG within the cavity. In previous work based on AIFG[
Figure 4.(a) Output power of the LP01 and LP11 modes from the fiber oscillator as a function of the pump power; (b) output spectra and corresponding beam profiles of different operation modes.
Power scaling of the intra-cavity mode conversion seed fiber laser by MOPA is also performed. The seed power of 7.22 W in the
Figure 5.(a) Output power of the LP01 and LP11 modes from the amplifier as a function of the pump power (insets: mode profiles of the LP11 mode under different pump powers); (b) output spectra of the LP01 and LP11 modes under the maximum output power.
The switching time between the
Figure 6.(a) Switching time between the LP01 and LP11 modes of the seed laser with the AIFG outside the fiber oscillator; (b) switching time between the LP01 and LP11 modes from the amplifier with the AIFG outside the fiber oscillator.
Figure 7.(a) Switching time between the LP01 and LP11 modes of the seed laser with the AIFG inside the fiber oscillator; (b) switching time between the LP01 and LP11 modes from the amplifier with the AIFG inside the fiber oscillator.
Figure 8.Output power of the LP11 mode in both configurations recorded in the total duration of 10 min.
The stability of the output power of the
In comparison, although similar efficiencies, output powers, and switching times are obtained from the power amplifiers with two distinctively different mode-switchable seed lasers, quite different characteristics are found in these two seed lasers. The seed laser with the AIFG outside the cavity is superior in terms of switching time and overall efficiency owing to a simpler mode conversion process and lower insertion loss, which makes it more advantageous as the seed laser. In addition, it is also worth studying the mode purity difference with the AIFG inside and outside the laser cavity, which will be carried out through mode decomposition in the near future. In fact, since the AIFG is a passive component, it is also possible to realize a high-power mode switchable fiber laser with output power up to hundreds and even thousands of watts by integrating an AIFG directly after a high-power fiber laser.
Moreover, the fiber laser system adopting the AIFG can potentially be applied in the study of transverse mode instability (TMI). The TMI is recognized as the mode coupling process between the fundamental mode and high-order modes on the kHz level[
4. Conclusion
In conclusion, we have demonstrated a high-power mode switchable fiber laser system based on an AIFG. Two kinds of several-watt-level mode switchable seed fiber lasers, with the AIFG inside and outside the laser cavity, are adopted as the seeds for power amplification. In comparison with all the previous intra-cavity mode switchable fiber lasers based on AIFG, a true few-mode fiber laser is realized with a designated setup, where the few-mode laser could extract the gain. Furthermore, by taking advantage of the MOPA technique, more than 100 W output power in both the
References
[1] Y. Feng, L. R. Taylor, D. B. Calia. 25 W Raman-fiber-amplifier-based 589 nm laser for laser guide star. Opt. Express, 17, 19021(2009).
[2] W. Shi, Q. Fang, X. Zhu, R. A. Norwood, N. Peyghambarian. Fiber lasers and their applications [Invited]. Appl. Opt., 53, 6554(2014).
[3] J. Zhao, G. Guiraud, C. Pierre, F. Floissat, A. Casanova, A. Hreibi, W. Chaibi, N. Traynor, J. Boullet, G. Santarelli. High-power all-fiber ultra-low noise laser. Appl. Phys. B, 124, 114(2018).
[4] J. Shi, C. Li, H. Mao, Y. Ren, Z.-C. Luo, A. Rosenthal, K. K. Y. Wong. Grüneisen-relaxation photoacoustic microscopy at 1.7 µm and its application in lipid imaging. Opt. Lett., 45, 3268(2020).
[5] R. Klas, A. Kirsche, M. Gebhardt, J. Buldt, H. Stark, S. Hädrich, J. Rothhardt, J. Limpert. Ultra-short-pulse high-average-power megahertz-repetition-rate coherent extreme-ultraviolet light source. PhotoniX, 2, 4(2021).
[6] D. J. Richardson, J. Nilsson, W. A. Clarkson. High power fiber lasers: current status and future perspectives. J. Opt. Soc. Am. B, 27, B63(2010).
[7] M. N. Zervas, C. A. Codemard. High power fiber lasers: a review. IEEE J. Sel. Top. Quantum Electron., 20, 219(2014).
[8] P. Zhou, L. Huang, J. Xu, P. Ma, R. Su, J. Wu, Z. Liu. High power linearly polarized fiber laser: generation, manipulation and application. Sci. China Technol. Sci., 60, 1784(2017).
[10] . Advanced metal processing–high-power fiber lasers with rogrammable beam technology.
[12] D. Mao, Y. Zheng, C. Zeng, H. Lu, C. Wang, H. Zhang, W. Zhang, T. Mei, J. Zhao. Generation of polarization and phase singular beams in fibers and fiber lasers. Adv. Photonics, 3, 014002(2021).
[13] J. M. O. Daniel, W. A. Clarkson. Rapid, electronically controllable transverse mode selection in a multimode fiber laser. Opt. Express, 21, 29442(2013).
[14] X. Heng, J. Gan, Z. Zhang, J. Li, M. Li, H. Zhao, Q. Qian, S. Xu, Z. Yang. Transverse mode switchable all-fiber Brillouin laser. Opt. Lett., 43, 4172(2018).
[15] N. Wang, J. C. Alvarado-Zacarias, M. S. Habib, H. Wen, J. E. Antonio-Lopez, P. Sillard, A. Amezcua-Correa, A. Schülzgen, R. Amezcua-Correa, G. Li. Mode-selective few-mode Brillouin fiber lasers based on intramodal and intermodal SBS. Opt. Lett., 45, 2323(2020).
[16] Y. Cai, J. Wang, J. Zhang, H. Wan, Z. Zhang, L. Zhang. Generation of cylindrical vector beams in a mode-locked fiber laser using a mode-selective coupler. Chin. Opt. Lett., 16, 010602(2018).
[17] T. Wang, A. Yang, F. Shi, Y. Huang, J. Wen, X. Zeng. High-order mode lasing in all-FMF laser cavities. Photonics Res., 7, 42(2018).
[18] J. Lu, L. Meng, F. Shi, X. Liu, Z. Luo, P. Yan, L. Huang, F. Pang, T. Wang, X. Zeng, P. Zhou. Dynamic mode-switchable optical vortex beams using acousto-optic mode converter. Opt. Lett., 43, 5841(2018).
[19] K. Wei, W. Zhang, L. Huang, D. Mao, F. Gao, T. Mei, J. Zhao. Generation of cylindrical vector beams and optical vortex by two acoustically induced fiber gratings with orthogonal vibration directions. Opt. Express, 25, 2733(2017).
[20] W. Zhang, K. Wei, H. Wang, D. Mao, F. Gao, L. Huang, T. Mei, J. Zhao. Tunable-wavelength picosecond vortex generation in fiber and its application in frequency-doubled vortex. J. Opt., 20, 014004(2017).
[21] W. Zhang, L. Zhang, C. Meng, F. Gao. Generation of nanosecond cylindrical vector beams in two-mode fiber and its applications of stimulated Raman scattering. Chin. Opt. Lett., 19, 010603(2021).
[22] R. Su, B. Yang, X. Xi, P. Zhou, X. Wang, Y. Ma, X. Xu, J. Chen. 500 W level MOPA laser with switchable output modes based on active control. Opt. Express, 25, 23275(2017).
[23] Y. You, G. Bai, X. Zou, X. Li, M. Su, H. Wang, Z. Quan, M. Liu, J. Zhang, Q. Li, H. Shen, Y. Qi, B. He, J. Zhou. A 1.4-kW mode-controllable fiber laser system. J. Lightwave Technol., 39, 2536(2021).
[24] X. Du, H. Zhang, P. Ma, X. Wang, P. Zhou, Z. Liu. Spatial mode switchable fiber laser based on FM-FBG and random distributed feedback. Laser Phys., 25, 095102(2015).
[25] Y. Cai, Z. Wang, H. Wan, Z. Zhang, L. Zhang. Mode and wavelength-switchable pulsed fiber laser with few-mode fiber grating. IEEE Photonics Technol. Lett., 31, 1155(2019).
[26] B. Sun, A. Wang, L. Xu, C. Gu, Z. Lin, H. Ming, Q. Zhan. Low-threshold single-wavelength all-fiber laser generating cylindrical vector beams using a few-mode fiber Bragg grating. Opt. Lett., 37, 464(2012).
[27] L. Huang, J. Leng, P. Zhou, S. Guo, H. Lu, X. Cheng. Adaptive mode control of a few-mode fiber by real-time mode decomposition. Opt. Express, 23, 28082(2015).
[28] D. Lin, J. Carpenter, Y. Feng, S. Jain, Y. Jung, Y. Feng, M. N. Zervas, D. J. Richardson. Reconfigurable structured light generation in a multicore fibre amplifier. Nat. Commun., 11, 3986(2020).
[29] J. Song, H. Xu, H. Wu, J. Ye, J. Xu, L. Huang, J. Leng, P. Zhou. All-fiberized transverse mode-switching method based on temperature control. Appl. Opt., 58, 3696(2019).
[30] C. Jocher, C. Jauregui, M. Becker, M. Rothhardt, J. Limpert, A. Tünnermann. An all-fiber Raman laser for cylindrical vector beam generation. Laser Phys. Lett., 10, 125108(2013).
[31] J. Lv, H. Li, Y. Zhang, R. Tao, Z. Dong, C. Gu, P. Yao, Y. Zhu, W. Chen, Q. Zhan, L. Xu. Few-mode random fiber laser with a switchable oscillating spatial mode. Opt. Express, 28, 38973(2020).
[32] H. Wu, J. Lu, L. Huang, X. Zeng, P. Zhou. All-fiber laser with agile mode-switching capability through intra-cavity conversion. IEEE Photonics J., 12, 1500709(2020).
[33] K. O. Hill, G. Meltz. Fiber Bragg grating technology fundamentals and overview. J. Lightwave Technol., 15, 1263(1997).
[34] K. J. Lee, I.-K. Hwang, H. C. Park, B. Y. Kim. Axial strain dependence of all-fiber acousto-optic tunable filters. Opt. Express, 17, 2348(2009).
[35] P. Ma, H. Xiao, D. Meng, W. Liu, R. Tao, J. Leng, Y. Ma, R. Su, P. Zhou, Z. Liu. High power all-fiberized and narrow-bandwidth MOPA system by tandem pumping strategy for thermally induced mode instability suppression. High Power Laser Sci. Eng., 6, e57(2018).
[36] L. Xie, C. Zhang, Y. Liu, H. Li, Q. Chu, H. Song, W. Wu, B. Shen, M. Li, X. Feng, S. Huang, R. Tao, J. Wang, X. Zhang, H. Zhu. Experimental investigation of quasi-static mode degradation in a high power large mode area fiber amplifier. Opt. Express, 29, 7986(2021).
[37] H.-J. Otto, C. Jauregui, F. Stutzki, F. Jansen, J. Limpert, A. Tünnermann. Controlling mode instabilities by dynamic mode excitation with an acousto-optic deflector. Opt. Express, 21, 17285(2013).
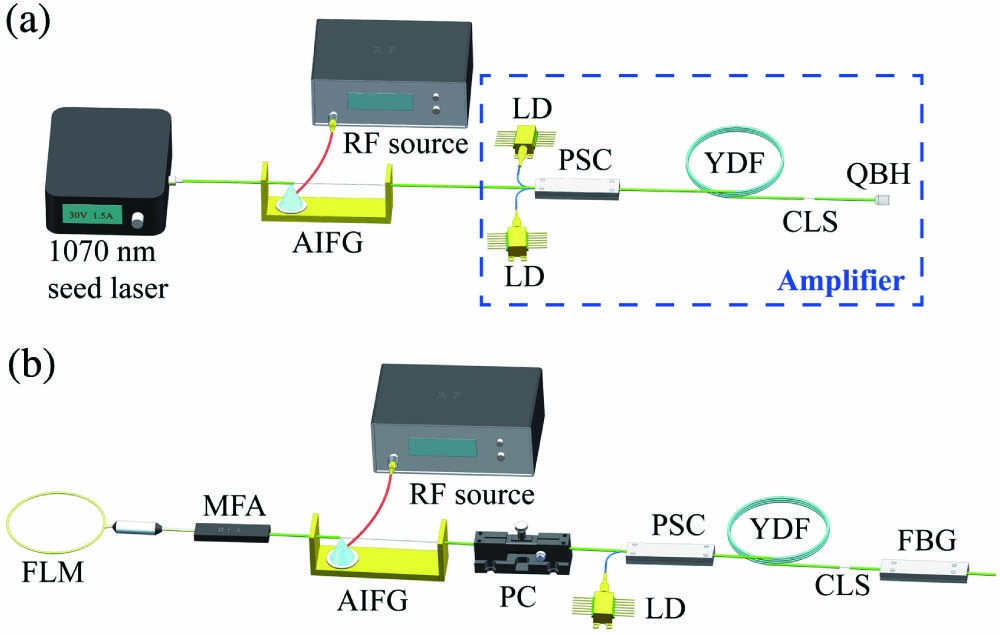
Set citation alerts for the article
Please enter your email address