Author Affiliations
Australian National University, Research School of Physics, Nonlinear Physics Center, Canberra, Australian Capital Territory, Australiashow less
Fig. 1. Resonances in metaphotonic structures. (a) SEM images of selected plasmonic metastructures (red) with LSPR, SLR, and BIC resonances, respectively. (Left) Figure adapted from Ref.
31, with permission from AIP Publishing; (middle) adapted with permission from Ref.
32 ©2010, American Physical Society (APS); (right) adapted with permission from Ref.
33 ©2020 American Chemical Society (ACS). (b) Types of resonances in plasmonic (red), dielectric (green), and both types of materials (red-green). These include LSPR, lattice mode (SLR and GMR), BIC, and Mie resonance. (c) SEM images of selected dielectric metastructures with GMR, BIC, and Mie resonances. (Left) Figure adapted with permission from Ref.
34 ©2013 ACS; (middle) adapted with permission from Ref.
35 ©2022 ACS; (right) adapted with permission from Ref.
36 ©2020 ACS.
Fig. 2. Nonlinear chiroptical response from nonresonant metallic metastructures. (a) Metasurface composed of a square array of L-shaped gold nanoparticles with small defects. Figure adapted with permission from Ref.
50 ©2006 IOP Publishing (all rights reserved). Top: SEM image; bottom: nonresonant SH signal spectrum dependence on the orientation angle of the input beam polarizer. (b) Metasurface composed of gold twisted-cross nanodimers with 3D chirality. Figure adapted with permission from Ref.
51 ©2011 Optica. Top: SEM images; bottom: nonresonant SH microscopy maps for left-handed nanodimers for LCP and RCP pump, respectively. (c) Metasurface composed of triangular nanoholes in gold arranged in a honeycomb lattice. Top: SEM image; bottom: polarization-resolved SH spectra in the visible range for the LCP excitation. Figure adapted with permission from Ref.
52 ©2014 APS.
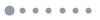
Fig. 3. Nonlinear chiroptical response from resonant plasmonic metasurfaces. (a) Plasmonic metasurface with LSPR resonances composed of supercells of gold G-shaped meta-atoms arranged in a square lattice with
rotational symmetry. Top: SEM image; bottom: SH signal dependence on the pump polarization and SH microscopy maps for LCP and RCP excitation. Figure adapted with permission from Ref.
60 ©2009 ACS. (b) Nonlinear metasurfaces supporting plasmonic lattice modes with engineered macroscopic
,
, and
rotational symmetry. Top: SEM image; center: mode field profiles; bottom: TH signal maps for LCP and RCP excitation for corresponding metasurfaces. Figure adapted with permission from Ref.
61 ©2014 APS. (c) Nonlinear Pancharatnam–Berry phase metasurface composed of gold crosses hosting LSPR resonances in the near-IR range. Top: SEM image; bottom: experimental diffraction patterns of TH signals for the metasurfaces with geometric phase of 90 deg. Figure adapted with permission from Ref.
62 ©2015 Springer Nature. (d) Integrated nonlinear metasurface supporting hybrid plasmonic-photonic modes. Top: SEM image; bottom: TGH intensity for LCP and RCP excitation depending on the period of plasmonic metasurface
. Figure adapted with permission from Ref.
63 ©2019 John Wiley and Sons.
Fig. 4. Nonlinear chiroptical response from resonant dielectric metastructures. (a) VUV THG from dielectric
photonic crystal membrane hosting GMR modes. Top: schematic; center: linear transmission for structured and unstructured film and SEM image; bottom: LCP and RCP components of the TH intensity for the RCP pump beam. Figure adapted with permission from Ref.
75 ©2020 Optica. (b) TH CD from Mie-resonant CdTe nanostructured helices. Top: schematic; center: measured linear ellipticity and calculated differential extinction cross-section spectra; bottom: TH intensity in the forward direction versus pump laser power for the LCP and RCP pump and SEM image. Figure adapted with permission from Ref.
76 ©2022 Springer Nature. (c) Nonlinear CD in Mie-resonant dimer by breaking symmetry. Top: schematic; center and bottom: measured SH intensity and SH CD for LCP and RCP pump. Figure adapted with permission from Ref.
77 ©2021 ACS.
Fig. 5. Nonlinear chiral high-
dielectric metasurfaces supporting quasi-BICs. (a) Chiral broken-symmetry nonlinear metasurface hosting a quasi-BIC mode. Left: schematic and SEM image; right: linear transmission and CD (upper) and TH intensity and TH CD (lower) for the LCP and RCP pump in the vicinity of the quasi-BIC resonance. Figure adapted with permission from Ref.
46 (CC-BY). (b) Asymmetric Si metasurface demonstrating record-high TH CD with strong THG efficiency via excitation of quasi-BICs and GMR mode. Left: schematic and SEM image; right: measured forward TH signal and TH CD for the optimized sample with
. Figure adapted with permission from Ref.
90 ©2023 ACS.