Abstract
Two-dimensional materials have shown great application potential in high-performance electronic devices because they are ultrathin, have an ultra-large specific surface area, high carrier mobility, efficient channel current regulation, and extraordinary integration. In addition to graphene, other types of 2D nanomaterials have also been studied and applied in photodetectors, solar cells, energy storage devices, and so on. Bi2O2Se is an emerging 2D semiconductor material with very high electron mobility, modest bandgap, near-ideal subthreshold swing, and excellent thermal and chemical stability. Even in a monolayer structure, Bi2O2Se has still exhibited efficient light absorption. In this mini review, the latest main research progresses on the preparation methods, electric structure, and the optical, mechanical, and thermoelectric properties of Bi2O2Se are summarized. The wide rang of applications in electronics and photoelectronic devices are then reviewed. This review concludes with a discussion of the existing open questions/challenges and future prospects for Bi2O2Se.Two-dimensional materials have shown great application potential in high-performance electronic devices because they are ultrathin, have an ultra-large specific surface area, high carrier mobility, efficient channel current regulation, and extraordinary integration. In addition to graphene, other types of 2D nanomaterials have also been studied and applied in photodetectors, solar cells, energy storage devices, and so on. Bi2O2Se is an emerging 2D semiconductor material with very high electron mobility, modest bandgap, near-ideal subthreshold swing, and excellent thermal and chemical stability. Even in a monolayer structure, Bi2O2Se has still exhibited efficient light absorption. In this mini review, the latest main research progresses on the preparation methods, electric structure, and the optical, mechanical, and thermoelectric properties of Bi2O2Se are summarized. The wide rang of applications in electronics and photoelectronic devices are then reviewed. This review concludes with a discussion of the existing open questions/challenges and future prospects for Bi2O2Se.1. Introduction
Two-dimensional (2D) materials have attracted much attention due to their ultrathin atomic layer thickness, tunable band structures, super large specific surface area, good mechanical flexibility, and rich physical and chemical properties. In the last decade, great progress has been made in the application of 2D materials in the next generation of electronics, optoelectronics[1–3], sensors[4–6], energy storage device[7], and flexible electronics[8]. As an allotrope of carbon, graphene is the earliest-found and most popular 2D material (Figs. 1(a)–1(c)). It has excellent electrical, thermal, optical, and mechanical properties, and therefore has wide application in the fields of information, energy, medical treatment, military, and so on[9]. However, as a leading member of the 2D material family, single-layer graphene has a zero-optical bandgap, which greatly limits its applications in semiconductor logic devices, storage, and photocatalysis. Moreover, the monolayer graphene only absorbs 2.3% of incident light power. Therefore, researchers have turned their attention to other 2D nanomaterials, such as black phosphorus[10–12] and transition metal chalcogenides (TMDCs)[13], and so on. Black phosphorus (Fig. 1(d)) has high carrier mobility (~1000 cm2/(V·s) at room temperature) and anisotropic mechanical and electrical transport properties; however, its environmental instability is challenging for its practical application in air. TMDCs (Fig. 1(e)) represent a large family with a chemical formula of MX2, where M is a transition metal atom and X is a chalcogen atom. Most TMDCs with atomically thickness exhibit direct bandgap, strong spin-orbit coupling, fairly high mobility, and favorable electronic and optical properties. Following the research upsurge of graphene, black phosphorous, and transition metal dichalcogenides, multiple-element layered 2D materials have become a new research focus due to their more abundant structure and more diversified characteristics. Therefore, it is interesting and significant to find ternary or even multi-element 2D materials and explore their possibility in future applications.
![(Color online) (a) Graphene optical photographs with a thickness of about 3 nm. (b) Atomic force microscope images of monolayer graphene. Reproduced with permission[9]. Copyright 2004, The American Association for the Advancement of Science. (c) Schematic diagram of the atomic structure of graphene. (d) Schematic diagram of black phosphorus atomic structure. Reproduced with permission[14]. Copyright 2014, Nature Publishing Group. (e) Schematic diagram of MoS2 atomic structure. Reproduced with permission[15]. Copyright 2011, Nature Publishing Group.](/Images/icon/loading.gif)
Figure 1.(Color online) (a) Graphene optical photographs with a thickness of about 3 nm. (b) Atomic force microscope images of monolayer graphene. Reproduced with permission[9]. Copyright 2004, The American Association for the Advancement of Science. (c) Schematic diagram of the atomic structure of graphene. (d) Schematic diagram of black phosphorus atomic structure. Reproduced with permission[14]. Copyright 2014, Nature Publishing Group. (e) Schematic diagram of MoS2 atomic structure. Reproduced with permission[15]. Copyright 2011, Nature Publishing Group.
Recently, Bi2O2Se, as a ternary layered Bi-based oxychalcogenide material semiconductor material, has attracted much attention. Bi2O2Se has a modest bandgap of 0.8 eV, high mobility (1.9 K, 29 000 cm2/(V·s), room temperature, 450 cm2/(V·s)) and good thermal and chemical stability[11]. Bi2O2Se possesses a tetragonal crystal structure, with and layer stacking alternatively. Due to its interlayered zipper-like structure, it exhibits unique characteristics[16]. In contrast to other van der Waals 2D layered materials, the layers of Bi2O2Se are connected by relatively strong electrostatic forces. Therefore, special exfoliation method or growth strategy is developed to fabricate large-area and atomically thick Bi2O2Se thin films. In view of these stable, unique, and excellent properties, Bi2O2Se can be used for promising applications in integrated circuits[17–19], optoelectronics[20–22], thermoelectrics[23], neuromorphic computing[24,25], and so on.
In this review, we will briefly summarize the recent research progress of Bi2O2Se. First, the preparation methods, optical, mechanical, and thermoelectric properties are summarized. Then the applications as photodetectors (transistors), energy storage devices, memristors, optical switches, and biomedical devices are then elaborated. Finally, the existing open questions and prospects of Bi2O2Se are presented.
2. Preparation methods
A controllable preparation process of large-area and high-quality 2D Bi2O2Se thin films is the prerequisite for the application of electronic devices. Generally, the preparation methods of 2D materials are mainly divided into two categories: the top-down method and the bottom-up method. The top-down method is to obtain 2D thin films by peeling from bulk materials by chemical or mechanical means, including mechanical cleavage method, electrochemical Li-intercalation and exfoliation, Li-intercalation and exfoliation with n-butyllithium, and so on[26–30]. The production of TMDCs monolayers is usually achieved by micromechanical exfoliation of large crystals from top to bottom[9]. In theory, the mechanical exfoliation method can obtain thin films with high-quality, high-purity, and uniform thickness, which can be used to prepare electronic devices. However, this method has low repeatability and cannot effectively control the thickness and size of thin films. To improve reproducibility and controllability, a bottom-up method is needed to synthesize high-quality and large-area 2D single-crystal thin films. So far, many methods have been used to prepare more stable and high-quality Bi2O2Se single crystal, such as chemical vapor deposition (CVD)[11,31–36], modified Bridgman method[22,37], wet chemical synthesis, hydrothermal reactions[27,38], and so on. The bottom-up method can be used to grow Bi2O2Se single crystal directly on the target substrate by precisely controlling the growth conditions. Therefore it has more freedom to optimize the growth and improve the film quality.Table 1 summarizes the preparation methods, growth conditions, and basic film characteristics for Bi2O2Se reported in the literature.
Method | Precursor,growth conditions | Domain size (μm) | Thickness (nm) | Mobility (cm2/(V·s)) | Ref. |
---|
CVD | Bi2Se3, Bi2O3, 600–640 °C, 400 Torr | ~200 | 2–4 layers | ~313 (300 K)–20660 (2 K) | [31] |
CVD | Se, Bi2O3, 680 °C, 400 Torr | ~250 | 4 layers | 410 (RT) | [32] |
CVD | Bi2Se3,Bi2O3, 580–650 °C, 100-400 Torr | >200 | 6.7 | ~450 (RT)–29000 (1.9 K) | [11] |
CVD | Bi2Se3, Bi2O3, 550–630 °C, 30 Pa | ~180 | 9.8 | 98 (300 K) | [33] |
CVD | Bi2Se3, Bi2O3, 620 °C, 350–400 Torr | ~100 | 5.2 | 107 | [34] |
CVD | Bi2Se3, Bi2O3, <670 °C | ~200 | 0.65 | ~262 (RT) | [35] |
CVD | Bi2Se3, Bi2O3, >670 °C | >1700 | 10.8 | – | [35] |
Reverse-flow CVD | Bi2O2Se powder, 760 °C, 400 mbar | ~750 | 13.7 | 1400 (RT) | [47] |
Modified Bridgman method | Bi2O3, Se, Bi powder | Bulk | Bulk | 2.8 × 105 (2 K) | [37] |
Hydrothermalmethod | C6H13BiN2O7·H2O, Na2O3Se and KOH | >2 | 4.7 | – | [48] |
Hydrothermalmethod | Na2SeO3, C6H13BiN2O7·H2O and KOH | >60 | 4.92 | 334.7 (RT) | [49] |
Solution-assisted method | Bi(NO3)3·5H2O, (CH2OH)2, 500 °C, 400 Torr | Continuous | 8.5 | 74 (RT) | [18] |
Table 0. Summary of preparation methods, growth conditions, and basic characteristics for Bi2O2Se.
2.1. CVD growth
Chemical vapor deposition (CVD) is a common bottom-up method to fabricate 2D materials, in which a variety of growth parameters, such as heating temperature, gas pressure, precursor concentration, gas flow rate and so on, are needed to be optimized to realize the growth of atomically thin 2D film[39]. In this process, gaseous compounds are formed first and transported by inert gas to the target substrate, as shown inFig. 2(a). High-quality Bi2O2Se single crystal thin films with adjustable size, controllable thickness, and excellent electronic properties can be prepared by CVD method[11,20,40–42]. In the early stages, Wuet al. and Liet al. synthesized 2D Bi2O2Se crystals with large area, high mobility, and thin film on mica ([KMg3(AlSi3O10)F2]) using Bi2O3 and Bi2Se3 as precursors as shown inFig. 2(b)[31,41]. It has been confirmed that the nucleation sites, thickness, domain sizes, and crystal phase transition of Bi2O2Se thin films can be well controlled by adjusting growth conditions (Figs. 2(c)–2(f)). For example, by controlling the temperature, planar and vertical Bi2O2Se nanosheets can be synthesized as shown inFigs. 2(g) and2(h). At lower temperatures, a kinetics-dominated growth was met. Precursors tend to get rid of the restriction of the substrate and grow into the vertical direction. At higher growth temperatures, only Bi2O2Se with the lateral layout was grown, which is a thermodynamics-controlled growth process due to higher migration rate of adatoms[41,43].
![(Color online) Preparation of two-dimensional films by CVD method. (a) CVD preparation diagram. Reproduced with permission[31]. Copyright 2017, American Chemical Society. (b) 2D Bi2O2Se crystal synthesized on mica. Reproduced with permission[11]. Copyright 2017, Nature Publishing Group. (c–f) Domain size and crystal phase transition of Bi2O2Se thin films. Reproduced with permission[31]. Copyright 2017, American Chemical Society. (g, h) SEM of both transverse Bi2O2Se and vertical triangular Bi2OxSe. Reproduced with permission41]. Copyright 2018, Wiley-VCH. (i) Improved preparation method. Reproduced with permission[32]. Copyright 2019, American Chemical Society. (j, k) Schematic of VS growth mechanism. Reproduced with permission[20]. Copyright 2019, Wiley-VCH. (l) SEM pictures of Bi2O2Se on STO. Reproduced with permission[19]. Copyright 2019, American Chemical Society. (m) Vertical growth of 2D Bi2O2Se films. Reproduced with permission[43]. Copyright 2019, Wiley-VCH.](/Images/icon/loading.gif)
Figure 2.(Color online) Preparation of two-dimensional films by CVD method. (a) CVD preparation diagram. Reproduced with permission[31]. Copyright 2017, American Chemical Society. (b) 2D Bi2O2Se crystal synthesized on mica. Reproduced with permission[11]. Copyright 2017, Nature Publishing Group. (c–f) Domain size and crystal phase transition of Bi2O2Se thin films. Reproduced with permission[31]. Copyright 2017, American Chemical Society. (g, h) SEM of both transverse Bi2O2Se and vertical triangular Bi2OxSe. Reproduced with permission41]. Copyright 2018, Wiley-VCH. (i) Improved preparation method. Reproduced with permission[32]. Copyright 2019, American Chemical Society. (j, k) Schematic of VS growth mechanism. Reproduced with permission[20]. Copyright 2019, Wiley-VCH. (l) SEM pictures of Bi2O2Se on STO. Reproduced with permission[19]. Copyright 2019, American Chemical Society. (m) Vertical growth of 2D Bi2O2Se films. Reproduced with permission[43]. Copyright 2019, Wiley-VCH.
Carrier concentration is one of the most important parameters for semiconductor materials. In field effect transistors, the low carrier concentration of the channel can induce good gating, which is very important for reducing the operating voltage to manufacture low-power digital devices. However, when using Bi2O3 and Bi2Se3 as precursors, Bi2Se3 is firstly decomposed according to Bi2Se3(s) = 2BiSe(g) + Se2(g), and the decomposition may change greatly after prolonged heating, which will adversely affect the synthesis of 2D Bi2O2Se crystals, resulting in higher residual carrier concentration.
To eliminate the possible side reactions, defects, or vacancies, Wuet al. synthesized Bi2O2Se crystals using Se element and Bi2O3 powder as precursors through a two-temperature zone heating system. The fabricated Bi2O2Se film has an ultralow residual carrier concentration of 1016 cm–3 and high Hall carrier mobility up to 410 cm2/(V·s) at room temperature (Fig. 2(i))[32]. Compared with the complex and changeable decomposition reactions of Bi2Se3, Se elements mainly volatilize into single Se2 molecules. The dual heating zone system can change the relative partial pressure of Se and Bi precursors by controlling the heating temperature of Se and Bi2O3 sources separately. Finally, by optimizing the growth conditions, the defects or vacancies that lead to the n-type conductivity of Bi2O2Se can be greatly reduced, thus reducing the carrier concentration by 2–3 orders of magnitude. In previous reports, the size of the synthesized Bi2O2Se flakes is mostly at the micron scale. Wuet al. developed a new self-limiting vapor-solid (VS) deposition method to achieve the growth of millimeter 2D Bi2O2Se thin films on a mica substrate, in which Bi2O2Se powder as the sole growth source was put in a tubular furnace under normal pressure as shown inFigs. 2(j) and2(k), and the Bi2O2Se powder can be synthesized by hydrothermal method or CVD method[20].
Because the Bi-O layers in Bi2O2Se are structurally compatible with many perovskite oxides, and it exhibits rich and interesting physical properties (e.g, ferroelectricity, magnetism, thermoelectricity, etc.). Tanet al. synthesized Bi2O2Se on perovskite oxide by the CVD method and studied the growth process as shown inFig. 2(l), which provides an alternative platform for studying new physical phenomena of oxide heterostructures[19].
Due to the good lattice matching and good thermal stability, mica substrate is commonly used in the CVD growth of 2D Bi2O2Se. However, thanks to the strong binding force between mica substrate and Bi2O2Se thin films, it is difficult to transfer Bi2O2Se thin films to the new substrate to prepare the devices for the following measurement. In the application of 2D Bi2O2Se to electronic devices, SiO2/Si substrate has usually been used as a support for 2D materials. In transmission electron microscopy (TEM) characterization, Bi2O2Se needs to be transferred to a copper grid. It also requires a safety transfer method that could minimize the damage to Bi2O2Se as small as possible and at the same time is convenient to manipulate. Fuet al. used Raman spectroscopy to demonstrate the inevitable damage to Bi2O2Se when using hydrofluoric acid (HF) in the wet transferring. The authors developed a polystyrene (PS)-assisted noncorrosive transfer method. PS was firstly spin-coated onto the surface of f-mica and then baked. Subsequently, the PS film together with Bi2O2Se was peeled away from the f-mica with the assistance of deionized (DI) water. After this unique transfer method, the performance of Bi2O2Se devices is greatly improved[34]. Khanet al. used a polydimethylsiloxane (PDMS) and poly(methyl methacrylate) (PMMA)-assisted method to transfer Bi2O2Se flakes grown on mica substrate synthesized by VS deposition. Nevertheless this method is only effective in detaching thick Bi2O2Se flakes from mica[20,44]. Chenet al. developed a method to transfer Bi2O2Se sheets using PDMS only, which is proved to be a more convenient and effective method to transfer thinner Bi2O2Se flakes[44].
Wuet al. introduced Bi2O3 as a seed layer and realized the vertical growth of 2D Bi2O2Se films on mica substrate by the CVD method, as shown inFig. 2(m)[43]. These vertically grown Bi2O2Se thin films can be easily and cleanly transferred to the target substrate. Zhanget al. proposed a simple, rapid, and extensible solution-assisted method to synthesize high-quality Bi2O2Se thin films on flexible muscovite substrates through the decomposition of Bi(NO3)3·5H2O precursor and the following selenization. By changing the rotation speed of precursor solution, the thickness of the Bi2O2Se thin films can be accurately controlled to a few atomic layers[18]. However, due to the strain caused by limited growth temperature and the softness of the substrate, the electronic property of flexible device performance is usually poorer than that on rigid substrates.
2.2. The modified Bridgman method
Bridgman invented the crucible descent method in 1925, which was called the Bridgman method. Later, Stockbarger developed this method and named it as the B-S method, which is one of the most commonly used methods for preparing large-size single crystals. The brief preparation process is as follows: the precursor materials needed for crystals growth are placed in a cylindrical crucible and slowly lowered. The furnace temperature is controlled slightly above the melting point of the material through a heating furnace with a certain temperature gradient. When the crucible passes through the heating zone, the materials in the crucible are melted. When the crucible continues to fall, the temperature at the bottom of the crucible first drops below the melting point and starts to crystallize, and the crystals continue to grow as the crucible falls down. This method has many advantages. First, the shape of the crystals grown depends on the crucible, and the shape of the crucible can be designed according to the needs. Second, it is suitable for growing large-size single crystals and multiple crystals. Finally, the growth method is simple and easy to operate, which is convenient for automation and industrialization. Xuet al. and Chenet al. successfully synthesized uniform and high-quality Bi2O2Se single crystals in a vacuum quartz tube using Bi2O3, Se, and Bi powders as precursors by a modified Bridgman method[22,37].
2.3. Hydrothermal method
The hydrothermal method is also known as a high-pressure solution method. This method uses high-temperature and high-pressure aqueous solutions to dissolve or react substances that are insoluble or difficult to dissolve in water under atmospheric conditions to form a dissolved product of the substance and then crystallize and grow after reaching a certain degree of supersaturation. The hydrothermal method has the characteristics of mild reaction conditions, convenient and simple operation, the synthesized crystals have few defects, high uniformity, and high purity[45]. Tianet al. synthesized Bi2O2Se crystals by hydrothermal method using deionized water, hydrazine hydrate (N2H4·H2O), NaOH, Se powder, Bi(NO3)3·5H2O, LiNO3 powder, and KNO3 powder as raw materials[46]. Chen[21] and Khanet al.[20] also synthesized bulk Bi2O2Se by hydrothermal method using deionized water, LiNO3, KNO3, Bi(NO3)3·5H2O, Se, and N2H4·H2O as precursors.
3. Properties and characterizations
3.1. Crystal structure
2D layered material Bi2O2Se is a typical bismuth-based oxychalcogenide material with a layered structure. As shown inFig. 3(a)[46], the 4-fold symmetric Bi2O2Se has a tetragonal crystal structure and belongs to I4/mmm space group ( =b = 3.8 Å,c = 12.16 Å), in which there are 10 atoms in the unit cell[37,50], and the monolayer thickness of Bi2O2Se is about 0.61 nm[32,33]. Wuet al. confirmed the single crystal structure and monolayer thickness of Bi2O2Se by high-resolution transmission electron microscopy (HRTEM)[31]. As shown inFigs. 3(b) and3(c), the obtained lattice spacing of 0.19, 0.28 nm, and the layer spacing along the [001] direction of 0.61 nm is consistent with the theoretical values. Bi, O and Se atoms located at Wyckoff positions of 4e (0,0,z), 4d (0,1/2,1/4) and 2a (0,0,0), respectively. Therefore, the only free atomic coordinate in the structure is thez coordinate of the Bi atom. In the crystal structure, Bi atoms and O atoms form Bi2O2 layers perpendicular to [001] direction, and Se atoms form atomic layers located between the Bi2O2 layers. Bi2O2Se has a repeating sequence of ...-(Bi2O2)1-Se1-(Bi2O2)2-Se2-... layers[37]. For the Bi2O2Se nanosheet, the distance between Bi and Se (3.18 Å) is much larger than the sum of the effective ion radius of Bi3+ (~1.03 Å) and Se2− (~1.98 Å) but is shorter than that in bulk Bi2O2Se (3.27 Å). However, the Bi–O bond length (2.37 Å) is longer than that in bulk Bi2O2Se (2.31 Å). The results indicate that in the Bi2O2Se nanosheet the Bi–O bond is weaker while the interaction between Bi and Se layers is stronger than that in the bulk, which is conducive to the stability of the Bi2O2Se nanosheet[46,51,52]. In addition, the 2D square lattice formed by Bi-Bi with a distance of 3.8 Å in the layer is structurally compatible with many perovskite oxides and their heterostructure shows rich and interesting physical characteristics (ferroelectricity, magnetism, and high-Tc superconductivity)[9]. Bi2O2Se had very high stability and no phase transition occurred below 30 GPa[53].
![(Color online) (a) Crystal structure of Bi2O2Se. Reproduced with permission[46]. Copyright 2018, Wiley-VCH. (b, c) HRTEM of Bi2O2Se. Reproduced with permission[31]. Copyright 2017, American Chemical Society.](/Images/icon/loading.gif)
Figure 3.(Color online) (a) Crystal structure of Bi2O2Se. Reproduced with permission[46]. Copyright 2018, Wiley-VCH. (b, c) HRTEM of Bi2O2Se. Reproduced with permission[31]. Copyright 2017, American Chemical Society.
3.2. Electronic structure and optical property
Unlike other 2D semiconductor materials, layered Bi2O2Se lacks the standard van der Waals gap[16]. Consequently, it can be cleaved along the Se plane and the atomic structure may be rearranged at the surface, indicating the existence of nonequilibrium electrons distributed between and. Wuet al. combined the first-principle calculations and angle-resolved photoemission spectroscopy (ARPES) measurement to study the band structure and state density of bulk Bi2O2Se (Fig. 4). The minimum value of the conduction band (CBM) and the maximum value of the valence band (VBM) are located at points Γ and X, respectively, revealing its indirect band gap. The bands near CBM are very steep, while those near VBM are relatively flat[54]. By fitting the conduction band, a very low in-plane electron effective massm* = 0.14 ± 0.02m0 was obtained (m0 is free electron mass), which is conducive to achieving ultrahigh electron mobility[11]. Since the CBM and VBM of Bi and Se elements are mainly composed of p-orbitals, layered Bi2O2Se crystals are considered to have strong spin-orbit interaction[46].
![(Color online) (a–c) ARPES of Bi2O2Se films. Reproduced with permission[11]. Copyright 2017, Nature Publishing Group. (d) Band structure of Bi2O2Se films and bulk. Reproduced with permission[16]. Copyright 2019, Nature Publishing Group.](/Images/icon/loading.gif)
Figure 4.(Color online) (a–c) ARPES of Bi2O2Se films. Reproduced with permission[11]. Copyright 2017, Nature Publishing Group. (d) Band structure of Bi2O2Se films and bulk. Reproduced with permission[16]. Copyright 2019, Nature Publishing Group.
Bi2O2Se is a semiconductor with a narrow bandgap (0.8 eV), which is particularly valuable for infrared optoelectronic devices. Chenet al. also conducted systematic theoretical and experimental studies on the electronic structures of Bi2O2Se and plotted the integral band structure of Bi2O2Se by combining scanning tunneling microscope (STM) and angle-resolved photoelectron spectroscopy (ARPES), the bandgap from both STM and ARPES exhibited excellent spatial uniformity and robustness[37]. However, although the crystal structure of Bi2O2Se did not change under the influence of high pressure 4 GPa, the electronic change occurred including the crossing and anti-crossing behaviors of the top and the second top valence bands at different locations of the Brillouin zone due to the gradual shortening and hardening of the long and weak Bi-Se bonds between layers[53].
Raman spectroscopy is a non-destructive characterization tool, which can provide important information about structure, crystallinity, strain and defects by probing specific molecular vibration modes. According to group theory, there are 10 vibration modes based on the I4/mmm space group of Bi2O2Se, among which A1g, B1g, and Eg modes are Raman active. Khanet al. conducted Raman characterization for Bi2O2Se films with different thicknesses (Fig. 5(a)), showing that A1g characteristic peak was located at ~159 cm−1, and its strength decreased with the decrease of the thickness of Bi2O2Se films[20]. No obvious Raman characteristic peaks were observed for the monolayer Bi2O2Se films. Chenget al. combined the theory and experiment to study the Raman spectrum of Bi2O2Se.Fig. 5(b) demonstrates the four Raman vibration modes of Bi2O2Se at the Γ-point are 159.89 (A1g), 364.02 (B1g), 67.99 (Eg1), and 428.68 cm–1 (Eg2). It can be seen inFig. 5(c) that the A1g and B1g modes correspond to the movement of Bi and O atoms along theZ axis of crystallography. The vibration of Bi and O atoms in theXY-plane can cause two sets of degenerate Eg modes[55]. Whether or not the Raman vibration modes in crystals can be observed in the experiment depends on their experimental structures and Raman tensors. For Bi2O2Se, it is difficult to measure 2D thin film onXZ orYZplane, so only two intrinsic Raman peaks (A1g and B1g) can be observed. However, in the experiment, Chenget al. and Pereiraet al. did not observe the characteristic peak of Bi2O2Se near 364 cm−1 (B1g) predicted by group theory and only observed the A1g model (about 159.2 cm−1). As shown inFig. 5(d), Pereiraet al. explained the missing vibration mode of B1g based on the plasmon-phonon coupling L– or L+ bands of B1g modes due to the high carrier concentration of n-type semiconductor[53,55], just like many other highly doped semiconductors. Chenget al. found that all Raman modes show redshifts under tensile strains and blueshifts under compressive strains. The Eg mode exhibits to be the most sensitive mode affected by the uniaxial strain[53]. Pereiraet al. observed the pressure evolution of the experimental low-frequency Raman-active A1g and Eg modes. They ascribed the occurrence of several vibrational modes especially above 11.3 GPa could be related to the creation of defects in the sample[55]. Therefore, Raman spectroscopy affords a convenient and rapid means to identify the stain or defects in atomically thin film.
![(Color online) (a) Raman spectra of Bi2O2Se films with different layers. Reproduced with permission[20]. Copyright 2019, Wiley-VCH. (b–d) Four Raman vibration modes of Bi2O2Se. Reproduced with permission[55]. Copyright 2018, American Chemical Society.](/Images/icon/loading.gif)
Figure 5.(Color online) (a) Raman spectra of Bi2O2Se films with different layers. Reproduced with permission[20]. Copyright 2019, Wiley-VCH. (b–d) Four Raman vibration modes of Bi2O2Se. Reproduced with permission[55]. Copyright 2018, American Chemical Society.
Light absorption is related to the band structure of semiconductors. It is helpful to study the evolution of the band structure and to extract the optical bandgap. Wuet al. made optical measurements on 2D Bi2O2Se crystals with different thicknesses grown by CVD. The blue shift of the optical absorption edges occurred as the thickness decreased (Fig. 6(a)), which indicates that the bandgap increased due to the quantum size effect. By fitting the bandgap, it was found that the optical band gap can be adjusted from 1.37 to 1.90 eV as the thickness of 2D Bi2O2Se crystals gradually changed to monolayer, as shown inFig. 6(b)[31]. Liuet al. compared the results of photoluminescence, light transmission, and transient absorption spectroscopy with the electronic structure calculated by first-principles calculations. Due to the transition between the conduction band and the valence band state in the Γ valley, the multilayer Bi2O2Se (13 nm) has a direct optical transition near 720 nm (1.7 eV), which is almost the same as the result of monolayer Bi2O2Se.Figs. 6(c) and6(d) indicate that the electronic structure of the Γ valley does not change significantly with the change in thickness[56].
![(Color online) (a, b) Transmittance and band gap of 2D Bi2O2Se films and bulk. Reproduced with permission[31]. Copyright 2017, American Chemical Society. (c) Peak differential reflection (blue symbols) and PL (red curve) of the 13 nm nanoplate as a function of the probe wavelength (upper panel) and its transmittance spectrum (lower panel). Reproduced with permission[56]. Copyright 2020, Wiley-VCH. (d) Peak differential reflection (blue symbols) and PL (red curve) of the monolayer as a function of the probe wavelength. Reproduced with permission[56]. Copyright 2020, Wiley-VCH.](/Images/icon/loading.gif)
Figure 6.(Color online) (a, b) Transmittance and band gap of 2D Bi2O2Se films and bulk. Reproduced with permission[31]. Copyright 2017, American Chemical Society. (c) Peak differential reflection (blue symbols) and PL (red curve) of the 13 nm nanoplate as a function of the probe wavelength (upper panel) and its transmittance spectrum (lower panel). Reproduced with permission[56]. Copyright 2020, Wiley-VCH. (d) Peak differential reflection (blue symbols) and PL (red curve) of the monolayer as a function of the probe wavelength. Reproduced with permission[56]. Copyright 2020, Wiley-VCH.
3.3. Mechanical property
Graphene has been proven to be one of the strongest materials ever made. The mechanical strength (stiffness) of Bi2O2Se is also especially important for its application in various flexible functional devices. Zhanget al. calculated the mechanical flexibility of monolayer Bi2O2Se with the first-principles method, the monolayer Bi2O2Se has a greater Poisson's ratio and lower in-plane stiffness than other 2D materials (such as MoS2 and graphene)[57]. In the process of characterizing the crystal structure and chemical composition for the synthesized Bi2O2Se crystal, the Bi2O2Se can be transferred by a polydimethylsiloxane (PDMS) and poly(methyl methacrylate) (PMMA)-assisted method onto a Cu grid for transmission electron microscope (TEM) examination, as shown inFig. 7(a)[20]. Liet al. and Zhanget al. repeatedly measured the photoelectric properties of the Bi2O2Se devices on a flexible substrate, and the photoelectric properties showed excellent stability[18,41]. In addition, Yinet al. prepared 2D flexible Bi2O2Se photodetectors and photodetector arrays on mica, Bi2O2Se photodetector arrays exhibited excellent photoelectric performance and stability on a substrate with strain bending up to 1% (Figs. 7(b) and7(d)). They showed a very stable light response within 5 weeks in the environment, proving that the 2D Bi2O2Se photodetectors can work on a flexible substrate[40]. Chenet al. experimentally obtained the mechanical properties of 2D Bi2O2Se using the nanoindentation method. Few-layer Bi2O2Se exhibits a large intrinsic stiffness of 18–23 GPa, Young’s modulus of 88.7 ± 14.4 GPa, and can withstand a high radial strain of more than 3%, demonstrating excellent flexibility[44]. The presence of strain in 2D materials can change the band structure, carrier mobility, and so on. The strain effect is very important to understand the performance of flexible electronics. In the Raman spectrum, the stretching strain causes the Raman mode softening and red shift, while the compression strain causes the mode hardening and blue shift. The degeneracy Eg modes of Bi2O2Se split under uniaxial strain and shear strain, and the frequency variation of the degeneracy modes are anisotropic under rotating uniaxial strain[55]. In short, the excellent flexibility and stability of Bi2O2Se thin films make it an ideal semiconductor material for flexible and wearable or printable electronic devices.
![(Color online) (a) TEM image of Bi2O2Se on a copper grid. Reproduced with permission[20]. Copyright 2019, Wiley-VCH. (b) Photograph of 2D Bi2O2Se photodetectors and arrays on mica. (c) Optical image of 3 × 5 multi-pixel array of 2D Bi2O2Se photodetectors. (d) Photocurrent of a 2D Bi2O2Se photodetector in air. Reproduced with permission[40]. Copyright 2018, Nature Publishing Group.](/Images/icon/loading.gif)
Figure 7.(Color online) (a) TEM image of Bi2O2Se on a copper grid. Reproduced with permission[20]. Copyright 2019, Wiley-VCH. (b) Photograph of 2D Bi2O2Se photodetectors and arrays on mica. (c) Optical image of 3 × 5 multi-pixel array of 2D Bi2O2Se photodetectors. (d) Photocurrent of a 2D Bi2O2Se photodetector in air. Reproduced with permission[40]. Copyright 2018, Nature Publishing Group.
3.4. Thermoelectric property
Bi2O2Se was initially investigated as a potential n-type thermoelectric material in its bulk (ceramic) form[58]. Zhanget al. measured the electrical conductivity, thermal conductivity, and Seebeck coefficient of Bi2O2Se (65μm) in the temperature range of 300–470 K as shown inFig. 8. The thermal conductivity is only 0.346 W/(m·K) at 300 K[59]. The thermoelectric properties of materials are determined by their dimensionless thermoelectric ZT (ZT =S2σT/κ,S is Seebeck coefficient,σ is electrical conductivity,T is temperature, andκ is thermal conductivity). Generally, a material with ZT > 1 is considered to be excellent thermoelectric material. According to the investigation, the ZT value of Bi2O2Se can reach 0.2 at 800 K[58]. However, theoretical studies have shown that the dimensionless thermoelectric ZT of p-doped Bi2O2Se can reach 1.42 (800 K) with the in-plane strain. This can be compared with Bi2Te3, which is one of the most widely used and best thermoelectric materials. Recently, Yuet al. reported that the ZT value of n-doped Bi2O2Se is as high as 3.35 at 800 K[60]. This is much higher than the ZT value (2.6) of SnSe at 923 K, which is known to be the most effective thermoelectric material[61].
![(a) Electrical conductivityσ of the Bi2O2Se film as a function of temperature. (b) Thermal conductivityκ of the Bi2O2Se rectangular block as a function of temperature. (c) Seebeck coefficientS of the Bi2O2Se film as a function of temperature. Reproduced with permission[59]. Copyright 2013, Elsevier.](/Images/icon/loading.gif)
Figure 8.(a) Electrical conductivityσ of the Bi2O2Se film as a function of temperature. (b) Thermal conductivityκ of the Bi2O2Se rectangular block as a function of temperature. (c) Seebeck coefficientS of the Bi2O2Se film as a function of temperature. Reproduced with permission[59]. Copyright 2013, Elsevier.
The thermal transport of 2D materials is a key factor in thermal management, nanometer electronic devices, and thermoelectric devices. Yanget al. studied the in-plane and interfacial heat transfer and energy dissipation of 2D Bi2O2Se by Raman spectroscopy. Due to the low phonon group velocity, large surface scattering, and strong anharmonicity of Bi2O2Se phonons, the in-plane thermal conductivity of Bi2O2Se thin films decreases with the decrease in thickness. When the thickness of Bi2O2Se thin film is 8 nm, the in-plane thermal conductivity of Bi2O2Se is as low as 0.926–0.18 W/(m·K), which is far lower than that of other 2D materials such as black phosphorus and MoS2. However, contrary to the in-plane thermal conductivity coefficient, Bi2O2Se thin films have larger interface binding energy, the thinner Bi2O2Se has a stronger heat dissipation ability to the substrate, so its interface thermal conductivity increases as the thin films thickness decrease, reaching 21 MW/(m·K) when the thickness of Bi2O2Se thin film is 8 nm[62]. Yanget al. exhibited the photo-bolometric effect in Bi2O2Se photodetectors, which is based on temperature-induced hot carrier generation by light heating[63].
4. Applications
As a new 2D layered material, Bi2O2Se has a unique crystal structure and novel electron transport properties. Studies have shown that Bi2O2Se has ultrahigh carrier mobility, tunable bandgap, excellent thermoelectric property, perfect chemical and thermal stability, and controllable doping concentration, which are very attractive characteristics for electronic and optical applications. In the following, we present a brief review on the recent progress of Bi2O2Se in photodetectors, energy storage, memristors, optical switches, and biomedicine (Fig. 9).
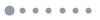
Figure 9.(Color online) Schematic representation of device applications of Bi2O2Se.
4.1. Photodetectors (transistors)
The mobility of semiconductors is one of the most important parameters, which determines some key performance of electronic devices. Wuet al. measured the Hall mobility of Bi2O2Se thin films at a low temperature (1.9 K) as high as 29 000 cm2/(V·s), which is comparable to graphene[11]. The top-gated Bi2O2Se (6.2 nm) field effect transistor (Fig. 10(a)) exhibited excellent performance at room temperature, including ultrahigh Hall mobility (up to 450 cm2/(V·s)), a high current on/off ratio (>106), and near-ideal subthreshold swing value (~65 mV/dec)[11]. In addition, the current on/off ratio and the Hall mobility of Bi2O2Se both changed with the thickness of thin films. As the channel thickness decreased, the value of the on/off ratio increased from ~103 to ~106. For thicker Bi2O2Se, the Hall mobility at room temperature remains almost constant. However, thanks to the Bi2O2Se thin films with a thickness of less than 6 nm, their Hall mobility would suddenly drop due to severe surface/interface scattering, as shown inFig. 10(b)[11]. Later, Tonget al. reported the hall mobility of Bi2O2Se thin film as 40 000 cm2/(V·s) at 2 K and attributed its ultrahigh mobility to the suppressed backscattering of electrons[33]. Since the mobility is closely related to the device performance such as photoconductive gain, response speed, and so on[64,65], it is significant to improve the mobility further by growth control, gate tuning, avoiding various scattering from the surface/interface, and surface encapsulation with a dielectric layer[66].
![(Color online) (a) Output curves of a 6.2 nm-thick Bi2O2Se device at room temperature. (b)μapp andIon/Ioff of Bi2O2Se FETs as a function of channel thickness. Reproduced with permission[11]. Copyright 2017, Nature Publishing Group. (c) OM image of centimeter-scale 2D Bi2O2Se arrays. (d) LinearIds–Vds curves of Bi2O2Se device with/without the illumination of 532 nm incident laser. Reproduced with permission[67]. Copyright 2017, Wiley-VCH. (e–g) Photocurrent, dark current, responsivity, detectivity, and response time as a function of temperature. Reproduced with permission[41]. Copyright 2018, Wiley-VCH.](/Images/icon/loading.gif)
Figure 10.(Color online) (a) Output curves of a 6.2 nm-thick Bi2O2Se device at room temperature. (b)μapp andIon/Ioff of Bi2O2Se FETs as a function of channel thickness. Reproduced with permission[11]. Copyright 2017, Nature Publishing Group. (c) OM image of centimeter-scale 2D Bi2O2Se arrays. (d) LinearIds–Vds curves of Bi2O2Se device with/without the illumination of 532 nm incident laser. Reproduced with permission[67]. Copyright 2017, Wiley-VCH. (e–g) Photocurrent, dark current, responsivity, detectivity, and response time as a function of temperature. Reproduced with permission[41]. Copyright 2018, Wiley-VCH.
The preparation of Bi2O2Se array is a prerequisite for fully exerting its potential in integrated optoelectronics and multi-pixel readout digital circuits. To this end, Wuet al. used diluted H2O2 and protonic mixed acid as an effective etching agent to accurately pattern the 2D semiconductor Bi2O2Se crystal with high mobility on mica and obtained a centimeter order 2D Bi2O2Se array. The etched 2D Bi2O2Se crystals still retain high carrier mobility of 209 cm2/(V·s) (room temperature), and the integrated photodetector of the prepared 2D Bi2O2Se arrays exhibited good air stability (After being exposed to the air for about 6 months, the performance of the device hardly changed, as shown inFig. 10(d)) and had a photoresponsivity up to 2000 A/W at 532 nm[67].
In recent decades, the near/medium infrared (IR) photodetectors have been widely used in military, academic, and commercial fields, and the semiconductor Bi2O2Se with a narrow bandgap of about 0.8 eV is particularly valuable for IR optoelectronic detection. Up to now, many people have reported on near/medium IR optoelectronic devices. First, Liet al. systematically studied the near-infrared photoelectric detection performance of Bi2O2Se thin films on mica substrate through variable temperature measurement. At 808 nm, the responsivity, detectivity, and response time reached 6.5 A/W, 8.3 × 1011 Jones and 2.8 ms, respectively[41]. When the temperature changed from 300 to 80 K, due to the thermal radiation of carriers at low temperatures being suppressed, the carrier density was reduced, resulting in a decrease of dark current from 152 to 1.5 nA. However, the photocurrent only changed slightly, and the photoelectric performance remained basically unchanged, as shown inFigs. 10(e)–10(g), indicating that the grown Bi2O2Se thin films had no surface trap states and shallow defect levels. In addition, there was no significant change in device performance when exposed to air for more than three months, demonstrating good air stability of the Bi2O2Se.
Most 2D layered materials, such as graphene, TMDCs, and so on, have not yet demonstrated both high sensitivity and rapid photoelectric response in infrared detection. However, the Bi2O2Se infrared photodetector on mica substrate reported by Yinet al. showed a high responsivity of 65 A/W at 1200 nm and an ultrafast response time of about 1 ps at room temperature[40]. At the same time, it exhibited a broadband optical response from visible light to 1700 nm. The photoelectric response reached 5800 A/W at 532 nm and 0.1 A/W at 1550 nm, which is comparable to other 2D materials such as graphene and TMDCs. In addition, 2D Bi2O2Se photodetectors can be integrated on flexible substrates and have a good imaging capability[40]. Due to the inherent indirect optical bandgap of Bi2O2Se, the photoresponse performance drops sharply at λ = 1550 nm (<0.1 A/W)[40]. To expand the Bi2O2Se response spectrum to the wider infrared, Luoet al.[68] used PbSe colloidal quantum dots (CQDs) to decorate Bi2O2Se to form a type II band structure, which facilitated the separation of photocarriers and improved the performance of Bi2O2Se photodetectors. Schematic diagrams of the hybrid photodetector under light excitation and band structure are shown inFigs. 11(a) and11(b), resulting in an optical response time of less than 4 ms and an infrared response greater than 103 A/W at 2μm, as shown inFigs. 11(c) and11(d).
![(Color online) (a) Schematic illustration of the PbSe/Bi2O2Se photodetector. (b) The estimated Type II energy band alignment between PbSe and Bi2O2Se before and after contact based on the estimated valence band offset and work function difference in UPS. (c) Photoresponse spectra. (d) Response decay dynamics of the hybrid photodetector. Reproduced with permission[68]. Copyright 2019, American Chemical Society. (e) Schematic illustration of the Bi2O2Se/MoSe2 heterojunction photodetector. Reproduced with permission[70]. Copyright 2019, Springer Science Business Media, LLC, part of Springer Nature. (f) OM image of as-fabricated device with 3-layered Bi2O2Se IPJ. (g) Output characteristic curve of the device in panel (m). Reproduced with permission[71]. Copyright 2019, Chinese Physical Society. (h) Preparation of Au/Bi2O2Se/Au MSM structures on mica substrates with a probe tip. Reproduced with permission[72]. Copyright 2019, Royal Society of Chemistry.](/Images/icon/loading.gif)
Figure 11.(Color online) (a) Schematic illustration of the PbSe/Bi2O2Se photodetector. (b) The estimated Type II energy band alignment between PbSe and Bi2O2Se before and after contact based on the estimated valence band offset and work function difference in UPS. (c) Photoresponse spectra. (d) Response decay dynamics of the hybrid photodetector. Reproduced with permission[68]. Copyright 2019, American Chemical Society. (e) Schematic illustration of the Bi2O2Se/MoSe2 heterojunction photodetector. Reproduced with permission[70]. Copyright 2019, Springer Science Business Media, LLC, part of Springer Nature. (f) OM image of as-fabricated device with 3-layered Bi2O2Se IPJ. (g) Output characteristic curve of the device in panel (m). Reproduced with permission[71]. Copyright 2019, Chinese Physical Society. (h) Preparation of Au/Bi2O2Se/Au MSM structures on mica substrates with a probe tip. Reproduced with permission[72]. Copyright 2019, Royal Society of Chemistry.
Compared with the monolayer or few-layer film, the multilayer film has higher state density and higher absolute light absorption, which can generate higher density photocurrent. Moreover, multilayer Bi2O2Se has a wider spectral response than few-layer Bi2O2Se due to the narrower bandgap. Yanget al. systematically studied the near-infrared photoelectric properties of multilayer Bi2O2Se thin films with a thickness of 30 nm, which had an ultra-sensitive optical response in the range of 850–1550 nm. The responsivity, detectivity, and external quantum efficiency reach 101 A/W, 1.9 × 1010 Jones, and 20 300% respectively at 1000 nm, and the response time is 30 ms (1500 nm)[69]. The results show that the multilayer Bi2O2Se has higher responsivity and external quantum efficiency than the few-layer Bi2O2Se reported in the literature[40], while maintaining a higher detectivity and a faster response time. Fu[34], Khan[20], and Tonget al.[33] investigated the performance of Bi2O2Se phototransistors in the UV–Visible–NIR spectrum, the maximum value of responsivity and detectivity reached 105 A/W and 1015 Jones, respectively. Yanget al.[70] fabricated Bi2O2Se-MoSe2 photodetector as shown inFig. 11(e). The heterostructure showed a detection range from visible light to near-infrared (405–808 nm) with response and detection efficiency of 413.1 mA/W and 3.79 × 1011 Jones at 780 nm, respectively.
To avoid defects or contaminants generated during traditional electrode deposition or sample transfer on devices, Honget al.[71] and Liuet al.[72] adopted different methods to improve the device structures. Honget al. synthesized Bi2O2Se thin films with different thicknesses on the steps of mica substrate to form in-plane homojunction. The device’s optical image is shown inFig. 11(f), and it exhibited a diode-like rectifying behavior with an on/off ratio of 102 (Fig. 11(g)). Maximum optical response of 2.5 A/W and a response time of 4.8μs are achieved. Liuet al. transferred the pre-deposited gold electrodes onto the Bi2O2Se thin films with a probe and prepared Au/Bi2O2Se/Au MSM structures on mica substrates to form metal/semiconductor contacts (Fig. 11(h)). Under optimized annealing temperature, the maximum responsivity and response time of the device reached 9.1 A/W and 36μs with a broadband spectral response ranging from UV to NIR (360–1090 nm). In the ultra-short channel, Yanget al. used the ab initio quantum transport simulation to predict the performance of Bi2O2Se FETs. The optimized n-type and p-type Bi2O2Se FET can meet or approach the high-performance requirements of the International Technology Roadmap for Semiconductors (ITRS)[17].Table 2 shows the comparisons of device performance of Bi2O2Se and other 2D materials.
Method | Precursor,growth conditions | Domain size (μm) | Thickness (nm) | Mobility (cm2/(V·s)) | Ref. |
---|
CVD | Bi2Se3, Bi2O3, 600–640 °C, 400 Torr | ~200 | 2–4 layers | ~313 (300 K)–20660 (2 K) | [31] |
CVD | Se, Bi2O3, 680 °C, 400 Torr | ~250 | 4 layers | 410 (RT) | [32] |
CVD | Bi2Se3,Bi2O3, 580–650 °C, 100-400 Torr | >200 | 6.7 | ~450 (RT)–29000 (1.9 K) | [11] |
CVD | Bi2Se3, Bi2O3, 550–630 °C, 30 Pa | ~180 | 9.8 | 98 (300 K) | [33] |
CVD | Bi2Se3, Bi2O3, 620 °C, 350–400 Torr | ~100 | 5.2 | 107 | [34] |
CVD | Bi2Se3, Bi2O3, <670 °C | ~200 | 0.65 | ~262 (RT) | [35] |
CVD | Bi2Se3, Bi2O3, >670 °C | >1700 | 10.8 | – | [35] |
Reverse-flow CVD | Bi2O2Se powder, 760 °C, 400 mbar | ~750 | 13.7 | 1400 (RT) | [47] |
Modified Bridgman method | Bi2O3, Se, Bi powder | Bulk | Bulk | 2.8 × 105 (2 K) | [37] |
Hydrothermalmethod | C6H13BiN2O7·H2O, Na2O3Se and KOH | >2 | 4.7 | – | [48] |
Hydrothermalmethod | Na2SeO3, C6H13BiN2O7·H2O and KOH | >60 | 4.92 | 334.7 (RT) | [49] |
Solution-assisted method | Bi(NO3)3·5H2O, (CH2OH)2, 500 °C, 400 Torr | Continuous | 8.5 | 74 (RT) | [18] |
Table 0. Summary of preparation methods, growth conditions, and basic characteristics for Bi2O2Se.
4.2. Energy storage devices
Polymer solar cells (PSCs) have attracted much attention because of their outstanding advantages such as simple structure and preparation process, low cost, light weight, and their ability to be made into flexible devices. Recently, some 2D materials have been applied to PSC. Huanget al. applied high-mobility Bi2O2Se thin films as active layers in PSCs to promote charge transfer[79]. The results show that the performance of the device has been significantly improved, the PCE of PBDB-T:ITIC-based device has increased from 10.09% (0% by weight) to 12.22% (2 wt%). The PCE of the PM6:Y6-based device reached 16.28% when 2 wt% Bi2O2Se is introduced. The optimized ternary device shows good air stability, indicating that the Bi2O2Se material has a good application prospect in photovoltaic devices (Fig. 12).
![(Color online) (a) Structure of polymer solar cells. (b) Energy diagram of the device. Reproduced with permission[79]. Copyright 2020, American Chemical Society.](/Images/icon/loading.gif)
Figure 12.(Color online) (a) Structure of polymer solar cells. (b) Energy diagram of the device. Reproduced with permission[79]. Copyright 2020, American Chemical Society.
4.3. Memristors
With the rapid expansion of data information, modern computers based on the von Neumann architecture are facing severe challenges. Intelligent computers that can learn, memorize and process information flexibly like the human brain are the direction and goal of future computer development. Memory resistors can remember their resistance history, which can be used on many occasions, such as nonvolatile storage devices, energy-efficient computers, neuromorphic calculating, and so on. Synapse refers to the part where neurons and neurons are connected which includes three parts: presynaptic membrane, synaptic cleft, and postsynaptic membrane[25,80–82]. The use of memristors to simulate synapses is based on the basic idea that the electrical properties can be altered under external stimuli and then memorized similar to synaptic plasticity, which makes it possible for memristors to build brain-like large-scale integrated circuits in the future.
Recently, Zhanget al. used the newly emerging 2D layered semiconductor material Bi2O2Se to realize a three-terminal memristor that simulates brain functions. Schematic diagram of the cross-sectional structure and the optical image are shown inFig. 13[25]. Zhanget al. demonstrated for the first time the coexistence of long-term plasticity (LTP) and short-term plasticity (STP) by decoupling the sites where the physical LTP and STP processes occurred. The concerted action of STP and LTP can make the transient synaptic efficacy from depression to facilitation be comprehensively adjusted through stimulus frequency or intensity. Through the heuristic recurrent neural circuitry model, the complex neural process of "sleep-wake cycle autoregulation" was simulated to show the complex computing power of memristors and the well-designed LTP and STP[25]. This work indicates that Bi2O2Se has great potential for complex neuromorphic functional devices for high dynamic neuromorphic computing. Furthermore, Yanget al.[24] developed a bidirectional all-optical synapse based on a 2D Bi2O2Se/Graphene hybrid structure. The hybrid structure presents both positive and negative photoresponsibility, which was used to realize all optically stimulated potentiation and depression. Recently, Yanet al. fabricate Bi2O2Se/PMN-PT 2D-FeFETs through growing high-quality Bi2O2Se epitaxial films on ferroelectric Pb(Mg1/3Nb2/3)O3-PbTiO3 (PMN-PT) single-crystal substrates. Thus, nonvolatile electric field modulation is achieved by changing the polarization direction of the ferroelectric layer, enabling the application of Bi2O2Se in nonvolatile memory[83].
![(Color online) (a) Schematic diagram of the Bi2O2Se device structure. (b) Optical image of the Bi2O2Se memristor. Reproduced with permission[25]. Copyright 2018, Wiley-VCH.](/Images/icon/loading.gif)
Figure 13.(Color online) (a) Schematic diagram of the Bi2O2Se device structure. (b) Optical image of the Bi2O2Se memristor. Reproduced with permission[25]. Copyright 2018, Wiley-VCH.
4.4. Optical switches
Pulsed lasers working in the mid-infrared (3–25µm) range have broad application prospects in the fields of national defense, military, biomedical research, environmental monitoring, sensing, atmospheric communications, and imaging. So far, nonlinear optical devices with the ability to generate pulsed lasers, called passive saturable absorbers or optical switches, are one of the key factors limiting the development of mid-infrared pulsed lasers. In particular, optical switches with broadband response can produce short pulse output by rapidly switching absorption[84]. Although graphene can be used as a saturable absorber near 3μm due to its zero bandgap, graphene has a low absorption coefficient (~2.3%) which limits its application in producing short-pulse lasers in the mid-infrared range. Therefore, finding new functional materials that can be used for mid-infrared short pulse generation is very important for the wide application of mid-infrared light sources.
Since layered Bi2O2Se can be split along its Se plane, the surface atomic structure may be rearranged. There are nonequilibrium electrons distribution between and layer. A planar topology system can be constructed through the Majorana bound state. Nonequilibrium electrons and potential energy topological states can excite optical nonlinearities. Tianet al. proved that Bi2O2Se as a saturable absorber has an ultra-wideband saturable nonlinear optical response in the wavelength range of 0.80 to 5.0μm through Z-scan technology and pump probe technology. At 5.0μm, the response time reaches the order of picoseconds and the response amplitude is as high as ~330.1%. An optical modulator based on 2D Bi2O2Se semiconductor can provide a simple, low-cost, effective, and scalable nonlinear absorption material for a 3µm Q-switched fiber laser.Fig. 14 shows a 3µm compact fiber laser that generates laser pulses through a Bi2O2Se-based optical switch[46]. Liet al. have applied optical switches to the field of terahertz systems. They used a PS-assisted transfer method to transfer Bi2O2Se to the silicon substrate to configure a Bi2O2Se/Si structured terahertz wave switch. The strong absorption of terahertz waves is caused by the accumulation of carriers at the interface. This device achieves an extinction ratio of 17.7 dB at an external laser irradiance of 1.3 W/cm2 at a broadband (0.25–1.5 THz) power density and also has a switching speed of 2 MHz. This shows that Bi2O2Se has potential for terahertz sensing, security, imaging, spectroscopy, communications, and so on[85].
![(Color online) Structure diagram of a Bi2O2Se optical switch. Reproduced with permission[46]. Copyright 2018, Wiley-VCH.](/Images/icon/loading.gif)
Figure 14.(Color online) Structure diagram of a Bi2O2Se optical switch. Reproduced with permission[46]. Copyright 2018, Wiley-VCH.
4.5. Biomedical applications
Photothermal technology has always attracted much attention in the field of cancer photothermal therapy (PTT). To improve the efficiency of treatment, researchers are committed to finding suitable materials with good photothermal properties and high tissue penetration ability under near-infrared (NIR) light irradiation[86–88]. In the past decade, 2D layered materials have been developed rapidly, which promoted the development of photothermal agents such as graphene oxide (GO), WS2, and MoS2. It is well known that Se is a low-toxicity therapeutic agent, and Bi is beneficial to the preparation of X-ray contrast agents with good biological tolerance.
Recently, Xieet al.[23] synthesized Bi2O2Se quantum dots (QDs) from bulk Bi2O2Se crystals by a simple solution method. TEM, AFM image, and cross-sectional analysis of the synthesized Bi2O2Se QDs are shown inFig. 15. Bi2O2Se QDs were used as photoacoustic (PA) imaging agents and photothermal therapy (PTT) reagents. It was confirmed that PA signal intensity increases with the increase of the concentration of QDs, and the smaller Bi2O2Se QDs had higher photothermal conversion efficiency. The photothermal conversion coefficient of Bi2O2Se QDs with size and thickness of 3.8 and 1.9 nm respectively is as high as 35.7%. Moreover, Bi2O2Se had a good photothermal stability. After four cycles of near-infrared laser irradiation, the temperature dropped only slightly (~1 °C). Experiments show that Bi2O2Se QDs have excellent PA performance and PTT efficiency. After the injection of the drug, QDs accumulated at the tumor site, making the PA imaging of the entire tumor clearer and stronger, which is conducive to imaging-guided PTT. The drug has no obvious toxicity. More importantly, Bi2O2Se QDs have an appropriate degradation rate in an aqueous solution, which can be almost completely degraded within 2 months. In contrast, the other two widely used inorganic PTT reagents, Au nanorods and GO nanosheets, both show high stability in aqueous solutions, indicating that they have poor degradability. Therefore, Bi2O2Se QDs have enough stability in the body to complete the treatment and will be discharged harmlessly from the body after treatment. The results show that the biodegradable Bi2O2Se QDs have promising application potential in imaging-guided PTT[23].
![(Color online) (a) TEM image, (b) AFM image, and (c) height analysis of Bi2O2Se QDs. Reproduced with permission[23]. Copyright 2019, Wiley-VCH.](/Images/icon/loading.gif)
Figure 15.(Color online) (a) TEM image, (b) AFM image, and (c) height analysis of Bi2O2Se QDs. Reproduced with permission[23]. Copyright 2019, Wiley-VCH.
5. Conclusion and outlook
In summary, we reviewed the recent research progress on Bi2O2Se. The review starts from the preparation method, the preparation conditions and growth characteristics. Then, the crystal structure, electronic structure, optical properties, mechanical properties, and thermoelectric performance of Bi2O2Se are introduced in detail. Subsequently, Bi2O2Se applications in optoelectronics, energy storage, neuromorphic computing, nonvolatile memory, terahertz, and biomedicine were presented.
2D Bi2O2Se has the characteristics of atomic layer thickness, ultrahigh mobility, moderate and tunable band structure, high chemical and thermal stability, and excellent mechanical flexibility. Therefore, it has quickly become a research focus since its first report by the Peng group. Its rich physical and chemical properties make Bi2O2Se a great promising application prospect in solar cells, energy storage, environmental catalysis, sensors, memory, and so on. Although Bi2O2Se is a new 2D material, we have seen rapid growth in research interest on it in recent years. However, there are still many challenges for its large-scale application in the future.
At present, 2D Bi2O2Se thin films have been synthesized generally by using a bottom-up method or top-down method. Nonetheless, the controllable preparation of 2D Bi2O2Se single crystals with large size and atomic thickness still needs further systematic study. Moreover, large-area transferring Bi2O2Se thin films without introducing extrinsic contamination or intrinsic defects still face tough challenges[34]. In photoelectric device applications, although QDs were used to improve the photoelectric sensitivity of Bi2O2Se photodetector, expensive production costs, complicated sensitization process, and even the heavy metal ions contained in QDs are great obstacles against putting Bi2O2Se into practical applications. In addition, the dark current of the device after sensitization becomes substantially higher, which is unfavorable for improving the device detectivity and the signal to noise ratio[68]. In an electronic Bi2O2Se thin film device, the heat generated in electric measurement is not easy to be dissipated in time due to its low in-plane thermal conductivity[62]. Therefore, overheating in electrical measurement burns the device. So the fine metal-semiconductor contact and heat management need more consideration.
The photodetectors (transistors), flexible electronic devices, energy storage devices, and so on that are described in this article are only part of the Bi2O2Se applications. The less studied and meaningful properties in 2D Bi2O2Se such as ferroelectricity and ferroelasticity, have great promise for future research in phase change engineering, piezoelectricity, electrostriction, and so on[89,90]. 2D Bi2O2Se has exhibited many excellent properties and has achieved some important applications in the fields of industrial production, environment monitoring, energy harvesting and medical treatment. However, the properties and application of Bi2O2Se still have a big room to be explored and more wonderful performance is expected in future research work.
References
[1] O Lopez-Sanchez, D Lembke, M Kayci et al. Ultrasensitive photodetectors based on monolayer MoS2. Nat Nanotechnol, 8, 497(2013).
[2] W J Zhang, J K Huang, C H Chen et al. High-gain phototransistors based on a CVD MoS2 monolayer. Adv Mater, 25, 3456(2013).
[3] Y H Chang, W J Zhang, Y H Zhu et al. Monolayer MoSe2 grown by chemical vapor deposition for fast photodetection. ACS Nano, 8, 8582(2014).
[4] C Anichini, W Czepa, D Pakulski et al. Chemical sensing with 2D materials. Chem Soc Rev, 47, 4860(2018).
[5] S P Xu, H X Fu, Y Tian et al. Exploiting two-dimensional Bi2O2Se for trace oxygen detection. Angew Chem Int Ed, 59, 17938(2020).
[6] K Shehzad, T J Shi, A Qadir et al. Environmental sensing: Designing an efficient multimode environmental sensor based on graphene-silicon heterojunction. Adv Mater Technol, 2, 1600262(2017).
[7] J F Li, Z Z Liang, Y F Wang et al. Enhanced efficiency of polymer solar cells through synergistic optimization of mobility and tuning donor alloys by adding high-mobility conjugated polymers. J Mater Chem C, 6, 11015(2018).
[8] Q A Vu, H Kim, V L Nguyen et al. Memristors: A high-on/off-ratio floating-gate memristor array on a flexible substrate via CVD-grown large-area 2D layer stacking. Adv Mater, 29, 1703363(2017).
[9] K S Novoselov, A K Geim, S V Morozov et al. Electric field effect in atomically thin carbon films. Science, 306, 666(2004).
[10] A Brown, S Rundqvist. Refinement of the crystal structure of black phosphorus. Acta Cryst, 19, 684(1965).
[11] J X Wu, H T Yuan, M M Meng et al. High electron mobility and quantum oscillations in non-encapsulated ultrathin semiconducting Bi2O2Se. Nat Nanotechnol, 12, 530(2017).
[12] Y T Zhao, H Y Wang, H Huang et al. Back cover: Surface coordination of black phosphorus for robust air and water stability (Angew. Chem. Int. Ed. 16/2016). Angew Chem Int Ed, 55, 5097(2016).
[13] K F Mak, C Lee, J Hone et al. Atomically thin MoS2: A new direct-gap semiconductor. Phys Rev Lett, 105, 136805(2010).
[14] L K Li, Y J Yu, G J Ye et al. Black phosphorus field-effect transistors. Nat Nanotechnol, 9, 372(2014).
[15] B Radisavljevic, A Radenovic, J Brivio et al. Single-layer MoS2 transistors. Nat Nanotechnol, 6, 147(2011).
[16] Q Wei, R Li, C Lin et al. Quasi-two-dimensional se-terminated bismuth oxychalcogenide (Bi2O2Se). ACS Nano, 13, 13439(2019).
[17] J Yang, R Quhe, Q Li et al. Sub 10 nm bilayer Bi2O2Se transistors. Adv Electron Mater, 5, 1800720(2019).
[18] C Zhang, J Wu, Y Sun et al. High-mobility flexible oxyselenide thin-film transistors prepared by a solution-assisted method. J Am Chem Soc, 142, 2726(2020).
[19] C Tan, M Tang, J Wu et al. Wafer-Scale growth of single-crystal 2D semiconductor on perovskite oxides for high-performance transistors. Nano Lett, 19, 2148(2019).
[20] U Khan, Y Luo, L Tang et al. Controlled vapor–solid deposition of millimeter-size single crystal 2D Bi2O2Se for high-performance phototransistors. Adv Funct Mater, 29, 1807979(2019).
[21] G Chen, J Wu, B Wang et al. High-performance self-powered photodetector based on Bi2O2Se nanosheets. Appl Phys A, 126, 579(2020).
[22] R Xu, S Wang, Y Li et al. Layered semiconductor Bi2O2Se for broadband pulse generation in the near-infrared. IEEE Photonics Technol Lett, 31, 1056(2019).
[23] H H Xie, M Q Liu, B H You et al. Photothermal therapy: Biodegradable Bi2O2Se quantum dots for photoacoustic imaging-guided cancer photothermal therapy. Small, 16, 2070013(2020).
[24] C Yang, T Chen, D Verma et al. Bidirectional all-optical synapses based on a 2D Bi2O2Se/graphene hybrid structure for multifunctional optoelectronics. Adv Funct Mater, 30, 2001598(2020).
[25] Z Y Zhang, T R Li, Y J Wu et al. Truly concomitant and independently expressed short-and long-term plasticity in a Bi2O2Se-based three-terminal memristor. Adv Mater, 31, 1805769(2019).
[26] M J Allen, V C Tung, R B Kaner. Honeycomb carbon: A review of graphene. Chem Rev, 110, 132(2010).
[27] X Huang, Z Y Zeng, H Zhang. Metal dichalcogenide nanosheets: Preparation, properties and applications. Chem Soc Rev, 42, 1934(2013).
[28] V Nicolosi, M Chhowalla, M G Kanatzidis et al. Liquid exfoliation of layered materials. Science, 340, 1226419(2013).
[29] Y Huang, Y H Pan, R Yang et al. Universal mechanical exfoliation of large-area 2D crystals. Nat Commun, 11, 2453(2020).
[30] Q Fu, J Q Dai, X Y Huang et al. One-step exfoliation method for plasmonic activation of large-area 2D crystals. Adv Sci, 2204247(2022).
[31] J X Wu, C W Tan, Z J Tan et al. Controlled synthesis of high-mobility atomically thin bismuth oxyselenide crystals. Nano Lett, 17, 3021(2017).
[32] J X Wu, C G Qiu, H X Fu et al. Low residual carrier concentration and high mobility in 2D semiconducting Bi2O2Se. Nano Lett, 19, 197(2019).
[33] T Tong, Y F Chen, S C Qin et al. Sensitive and ultrabroadband phototransistor based on two-dimensional Bi2O2Se nanosheets. Adv Funct Mater, 29, 1905806(2019).
[34] Q D Fu, C Zhu, X X Zhao et al. Ultrasensitive 2D Bi2O2Se phototransistors on silicon substrates. Adv Mater, 31, 1804945(2019).
[35] U Khan, L Tang, B F Ding et al. Nanoribbons: Catalyst-free growth of atomically thin Bi2O2Se nanoribbons for high-performance electronics and optoelectronics. Adv Funct Mater, 31, 2170230(2021).
[36] N Avishan, N Hussain, F Nosheen. Large-scale graphene production and transfer for industrial applications. MaterInnov, 2, 15(2022).
[37] C Chen, M X Wang, J X Wu et al. Electronic structures and unusually robust bandgap in an ultrahigh-mobility layered oxide semiconductor, Bi2O2Se. Sci Adv, 4, eaat8355(2018).
[38] T Zeng, Y C You, X F Wang et al. Chemical vapor deposition and device application of two-dimensional molybdenum disulfide-based atomic crystals. Prog Chem, 28, 459(2016).
[39] J X Yu, J Li, W F Zhang et al. Synthesis of high quality two-dimensional materialsvia chemical vapor deposition. Chem Sci, 6, 6705(2015).
[40] J B Yin, Z J Tan, H Hong et al. Ultrafast and highly sensitive infrared photodetectors based on two-dimensional oxyselenide crystals. Nat Commun, 9, 3311(2018).
[41] J Li, Z X Wang, Y Wen et al. High-performance near-infrared photodetector based on ultrathin Bi2O2Se nanosheets. Adv Funct Mater, 28, 1706437(2018).
[42] H X Fu, J X Wu, H L Peng et al. Self-modulation doping effect in the high-mobility layered semiconductor Bi2O2Se. Phys Rev B, 97, 241203(2018).
[43] Z Wu, G L Liu, Y X Wang et al. Seed-induced vertical growth of 2D Bi2O2Se nanoplates by chemical vapor transport. Adv Funct Mater, 29, 1906639(2019).
[44] W J Chen, U Khan, S M Feng et al. High-fidelity transfer of 2D Bi2O2Se and its mechanical properties. Adv Funct Mater, 30, 2004960(2020).
[45] X Zhang, Y F Liu, G H Zhang et al. Thermal decomposition of bismuth oxysulfide from photoelectric Bi2O2S to superconducting Bi4O4S3. ACS Appl Mater Interfaces, 7, 4442(2015).
[46] X L Tian, H Y Luo, R F Wei et al. Pulsed lasers: An ultrabroadband mid-infrared pulsed optical switch employing solution-processed bismuth oxyselenide. Adv Mater, 30, 1870233(2018).
[47] X Yang, Q Zhang, Y C Song et al. High mobility two-dimensional bismuth oxyselenide single crystals with large grain size grown by reverse-flow chemical vapor deposition. ACS Appl Mater Interfaces, 13, 49153(2021).
[48] M Q Li, L Y Dang l, G G Wang et al. Bismuth oxychalcogenide nanosheet: Facile synthesis, characterization, and photodetector application. Adv Mater Technol, 5, 2000180(2020).
[49] L Y Dang, M Q Liu, G G Wang et al. Organic ion template-guided solution growth of ultrathin bismuth oxyselenide with tunable electronic properties for optoelectronic applications. Adv Funct Mater, 32, 2201020(2022).
[50] T Tong, M H Zhang, Y Q Chen et al. Ultrahigh Hall mobility and suppressed backward scattering in layered semiconductor Bi2O2Se. Appl Phys Lett, 113, 072106(2018).
[51] M L Williams. CRC handbook of chemistry and physics. 76th ed. Occup Environ Med, 53, 504(1996).
[52] J Horák, Z Stary, P Lošťák et al. Anti-site defects in n-Bi2Se3 crystals. J Phys Chem Solids, 51, 1353(1990).
[53] A L J Pereira, D Santamaría-Pérez, R Vilaplana et al. Experimental and theoretical study of SbPO4 under compression. Inorg Chem, 59, 287(2020).
[54] X Pan, H X Chen, L Lu et al. Incorporating guanidinium as perovskitizer-cation of two-dimensional metal halide for crystal-array photodetectors. Chem Asian J, 16, 1925(2021).
[55] T Cheng, C W Tan, S Q Zhang et al. Raman spectra and strain effects in bismuth oxychalcogenides. J Phys Chem C, 122, 19970(2018).
[56] S Y Liu, C W Tan, D W He et al. Optical properties and photocarrier dynamics of Bi2O2Se monolayer and nanoplates. Adv Optical Mater, 8, 1901567(2020).
[57] Y Zhang, Q Gao, X Y Han et al. Mechanical flexibility and strain engineered-band structures of monolayer Bi2O2Se. Phys E, 116, 113728(2020).
[58] D L Guo, C G Hu, Y Xi et al. Strain effects to optimize thermoelectric properties of doped Bi2O2Se via tran-blaha modified becke-Johnson density functional theory. J Phys Chem C, 117, 21597(2013).
[59] K Y Zhang, C G Hu, X L Kang et al. Synthesis and thermoelectric properties of Bi2O2Se nanosheets. Mater Res Bull, 48, 3968(2013).
[60] J B Yu, Q Sun. Bi2O2Se nanosheet: An excellent high-temperature n-type thermoelectric material. Appl Phys Lett, 112, 053901(2018).
[61] L D Zhao, S H Lo, Y S Zhang et al. Ultralow thermal conductivity and high thermoelectric figure of merit in SnSe crystals. Nature, 508, 373(2014).
[62] F Yang, R D Wang, W W Zhao et al. Thermal transport and energy dissipation in two-dimensional Bi2O2Se. Appl Phys Lett, 115, 193103(2019).
[63] H Yang, C W Tan, C Y Deng et al. Bolometric effect in Bi2O2Se photodetectors. Small, 15, e1904482(2019).
[64] G Konstantatos, M Badioli, L Gaudreau et al. Hybrid graphene–quantum dot phototransistors with ultrahigh gain. Nat Nanotechnol, 7, 363(2012).
[65] W Liu, J H Lv, L Peng et al. Graphene charge-injection photodetectors. Nat Electron, 5, 281(2022).
[66] L Wang, I Meric, P Y Huang et al. One-dimensional electrical contact to a two-dimensional material. Science, 342, 614(2013).
[67] J Wu, Y Liu, Z Tan et al. Chemical patterning of high-mobility semiconducting 2D Bi2O2Se crystals for integrated optoelectronic devices. Adv Mater, 29, 1704060(2017).
[68] P Luo, F W Zhuge, F K Wang et al. PbSe quantum dots sensitized high-mobility Bi2O2Se nanosheets for high-performance and broadband photodetection beyond 2 μm. ACS Nano, 13, 9028(2019).
[69] H Yang, W Chen, X M Zheng et al. Near-infrared photoelectric properties of multilayer Bi2O2Se nanofilms. Nanoscale Res Lett, 14, 371(2019).
[70] T Yang, X Li, L M Wang et al. Broadband photodetection of 2D Bi2O2Se-MoSe2 heterostructure. J Mater Sci, 54, 14742(2019).
[71] C Y Hong, G F Huang, W W Yao et al. Thickness-modulated in-plane Bi2O2Se homojunctions for ultrafast high-performance photodetectors. Chin Phys B, 28, 367(2019).
[72] X L Liu, R P Li, C Y Hong et al. Highly efficient broadband photodetectors based on lithography-free Au/Bi2O2Se/Au heterostructures. Nanoscale, 11, 20707(2019).
[73] J Li, Z X Wang, J W Chu et al. Oriented layered Bi2O2Se nanowire arrays for ultrasensitive photodetectors. Appl Phys Lett, 114, 151104(2019).
[74] D Y Li, X Han, G Y Xu et al. Bi2O2Se photoconductive detector with low power consumption and high sensitivity. Acta Phys Sin Chin Ed, 69, 248502(2020).
[75] W Han, C Li, S J Yang et al. Atomically thin oxyhalide solar-blind photodetectors. Small, 16, e2000228(2020).
[76] M S Long, Z Shen, R J Wang et al. Ultrasensitive solar-blind ultraviolet photodetector based on FePSe3/MoS2 heterostructure response to 10.6 µm. Adv Funct Mater, 32, 2204230(2022).
[77] X Han, J Xing, H Xu et al. Remarkable improved photoelectric performance of SnS2 field-effect transistor with Au plasmonic nanostructures. Nanotechnology, 31, 215201(2020).
[78] Y Xu, A Ali, K Shehzad et al. Photodetectors: Solvent-based soft-patterning of graphene lateral heterostructures for broadband high-speed metal-semiconductor-metal photodetectors. Adv Mater Technol, 2, 1600241(2017).
[79] C W Huang, H Yu. 2D Bi2O2Se with high mobility for high performance polymer solar cells. ACS Appl Mater Interfaces, 12, 19643(2020).
[80] G L Ding, B D Yang, R S Chen et al. Reconfigurable 2D WSe2-based memtransistor for mimicking homosynaptic and heterosynaptic plasticity. Small, 17, 2170213(2021).
[81] J Y Du, C Ge, H Riahi et al. Dual-gated MoS2 transistors for synaptic and programmable logic functions. Adv Electron Mater, 6, 1901408(2020).
[82] Q Y Yang, Z D Luo, D W Zhang et al. Controlled optoelectronic response in van der Waals heterostructures for In-sensor computing. Adv Funct Mater, 32, 202207290(2022).
[83] J M Yan, J S Ying, M Y Yan et al. Optoelectronic coincidence detection with two-dimensional Bi2O2Se ferroelectric field-effect transistors. Adv Funct Mater, 31, 2103982(2021).
[84] F K Wang, S J Yang, J Wu et al. Emerging two-dimensional bismuth oxychalcogenides for electronics and optoelectronics. InfoMat, 3, 1251(2021).
[85] Z W Li, J S Li. Bi2O2Se for broadband terahertz wave switching. Appl Opt, 59, 11076(2020).
[86] X D Meng, Z Q Liu, Y Cao et al. Cancer therapy: Fabricating aptamer-conjugated PEGylated-MoS2/Cu1.8S theranostic nanoplatform for multiplexed imaging diagnosis and chemo-photothermal therapy of cancer. Adv Funct Mater, 27, 1605592(2017).
[87] Z Chen, P F Zhao, Z Y Luo et al. Cancer cell membrane-biomimetic nanoparticles for homologous-targeting dual-modal imaging and photothermal therapy. ACS Nano, 10, 10049(2016).
[88] B Li, F K Yuan, G J He et al. Ultrasmall CuCo2S4 nanocrystals: All-in-one theragnosis nanoplatform with magnetic resonance/near-infrared imaging for efficiently photothermal therapy of tumors. Adv Funct Mater, 27, 1606218(2017).
[89] M H Wu, X C Zeng. Bismuth oxychalcogenides: A new class of ferroelectric/ferroelastic materials with ultra high mobility. Nano Lett, 17, 6309(2017).
[90] Z Y Zhu, X P Yao, S Zhao et al. Giant modulation of the electron mobility in semiconductor Bi2O2Se via incipient ferroelectric phase transition. J Am Chem Soc, 144, 4541(2022).