
- Acta Photonica Sinica
- Vol. 50, Issue 5, 151 (2021)
Abstract
Keywords
0 Introduction
Optical Propagation Loss (OPL) is the core problem of Silicon-On-Insulator (SOI) optical waveguide devices, and the main source of the OPL is the inevitable Sidewall Roughness (SWR) caused by the waveguide fabrication processing [
The currently accepted theoretical model for the relationship between the waveguide SWR and the light guided mode is the PL theoretical model that was proposed by PAYNE F P and LACEY J P R in 1994 [
In this article, the improved theoretical model of defining the OPL coefficient on the SWR is exploited where the components of the SOI waveguide SWR at horizontal and vertical direction are introduced. Then, the measured values of 3-D distributed waveguide SWR are obtained with the CLSM technique. As a result, with such a combinative model, the dependences of the optical propagation loss coefficient of the waveguide on the SWR distribution and the width are systematically simulated. Finally, the great agreements between the numerical simulation results based on the measured SWR values and the experimental results are achieved.
1 Device structure and concepts
1.1 Device concepts and theoretical models for the OPL caused by SWR
Fig. 1 shows a schematic 3-D configuration of a straight waveguide in which the waveguide core has a refractive index of
Figure 1.Perspective view of a schematic SOI waveguide
where
Consequently, for both the TE-like and TM-like modes, the SWR induced optical scattering loss is related to both the y- and z-components of roughness, so the dependences of the optical intensity loss coefficients
where
and
where transferring functions,
1.2 Determination of the Correlation Length
The numerical simulation for the function

2 Numerical simulations of optical propagation loss
2.1 Numerical simulations for variables x and γ of the function
With Eq. (4), the numerical simulation results of two key variables

2.2 Numerical simulations for the correlation length
With the simulation results of

Note that the dependences of
3 Measurements for the anisotropic SWR and the OPL
3.1 Measurements for the SWR distributions of SOI waveguides
With the fabricated device sample, the reconstructed images of CLSM measurements for an SOI waveguide sidewall roughness could further be obtained. During measuring the SWR distributions, the chip of SOI waveguides is rotated by a quasi-90° angle and then measured the sidewall profile of the waveguide with CLSM. As a result, the average horizontal and the average vertical values of SWR are 22 nm and 23 nm, respectively. As defined in our previous work, the SWR values at both TE- and TM-mode are measured. In this definition of roughness, the
For the waveguide structure of fabricated device sample, with the theoretical model defined by Eqs. (1)~(7), the dependences of OPL coefficient on the SWR for both TE- and TM-mode are simulated as shown in Fig. 5. Note that the OPL coefficient caused by the SWR quickly increases with SWR and the TE-mode always bigger than the TM-mode. For instance, at the above measured values, 22 nm and 23 nm for the horizontal and vertical distributions, the OPL values for both the TE- and TM-mode are 5.0 dB/cm. So, a high-quality SOI waveguide, the SWR should be controlled lower than 20 nm. In addition, the difference of the OPL values between the TE- and TM-mode also quickly increases.
Figure 5.Numerical simulations for the OPL coefficient α3D vs SWR of SOI waveguide for TE- and TM-mode
In experiments for verifying the mean OPL value, the two ends of the waveguide chip are polished to form a Fabry-Perot (F-P) cavity. Then, a response of the optical resonance output to the temperature change is obtained as shown in Fig. 6. Since the F-P response curve to temperature change contains two wave periods, so, as labelled in Fig. 6, two periods: Period-1 and Period-2 are used to calculate the OPL values with the popular format as defined by Eq. (8)[
Figure 6.Propagation losses test of three SOI waveguides and the responses of F-P cavity formed by the waveguide channel to temperature change
where lwg represents the length of the waveguide [
4 Conclusion
As a conclusion, through self-consistent modeling and numerical simulations of the two key factors in the guided mode of an SOI waveguide: the correlation length and the SWR, the ideal simulation results are obtained. Based on the research on the surface roughness of SOI waveguides, we can introduce the 3-D distribution of SWR and the interaction effect between the optical field of guided mode and the SWR to create accurate theoretical model for defining the optical loss coefficient dependence on the waveguide structure and SWR.
References
[1] H YNAMAZAKI, T SAIDA et al. Dual carrier dual-polarization IQ modulator using complementary frequency shifter. IEEE Selected Topics on Quantum Electronics, 19, 3400208(2013).
[2] A S LIU, R JOHNS, L LIAO et al. A high-speed silicon optical modulator based on a metal-oxide-semiconductor capacitor. Nature, 427, 615-620(2004).
[3] H P SHANG, D G SUN, P YU et al. Investigation for sidewall roughness caused optical scattering loss of silicon-on-insulator waveguides with confocal laser scanning microscopy. Coatings, 10, 236(2020).
[4] F P PAYNE, J P R LACEY. A theoretical analysis of scattering loss from planar optical waveguides. Optical and Quantum Electronics, 26, 977-986(1994).
[5] T BARWICZ, H A HAUS. Three-dimensional analysis of scattering loss due to sidewall roughness in micro photonic waveguides. IEEE Lightwwave Technology, 23, 2719-2732(2005).
[6] C G POULTON, C KOOS, M FUJII et al. Radiation models and roughness loss in high index-contrast waveguides. IEEE Selected Topics on Quantum Electronics, 12, 1306-1321(2007).
[7] J H SCHMID, A DELÂGE, B LAMONTAGNE et al. Interference effect in scattering loss of high-index-contrast planar waveguides caused by boundary reflections. Optics Letters, 33, 1479-1481(2008).
[8] A DELÂGE, J LAPOINTE et al. Correlation of scattering loss, sidewall roughness and waveguide width in silicon-on-insulator (SOI) ridge waveguides. IEEE Journal of Lightwave Technology, 27, 3999-4008(2009).
[9] D G SUN, H P SHANG, H L JIANG et al. Effective metrology and standard of the surface roughness of micro/nanoscale waveguides with confocal laser scanning microscopy. Optics Letters, 44, 747-750(2019).
[10] H P SHANG, D G SUN, Q Y SUN et al. Analysis for system errors in measuring sidewall angle of silica waveguides with confocal laser scanning microscope (CLSM). Measurement Science and Technology, 30, 025004(2019).
[11] C R DOERR, K OKAMOTO. Planar lightwave circuits in fiber-optic communications. Optical Fiber Telecommunications, 269-341(2008).
[12] E J MURPHY. Optical circuits and components: design and applications(1999).
[13] C FEUCHTER T THIRSTRUP. High precision planar waveguide propagation loss measurement technique using a Fabry-Perot cavity. IEEE Photonics Technology Letters, 6, 1244-1247(1994).
[14] V A VLASOV, S J MCNAB. Losses in single-mode silicon-on-insulator strip waveguides and bends. Optics Express, 12, 1622-1631(2004).
[15] F BOEUF, S CREMER, E TEMPORITI. Silicon photonic R&D and manufacturing on 300-mm wafer platform. IEEE Lightwave Technology, 33, 286-295(2016).
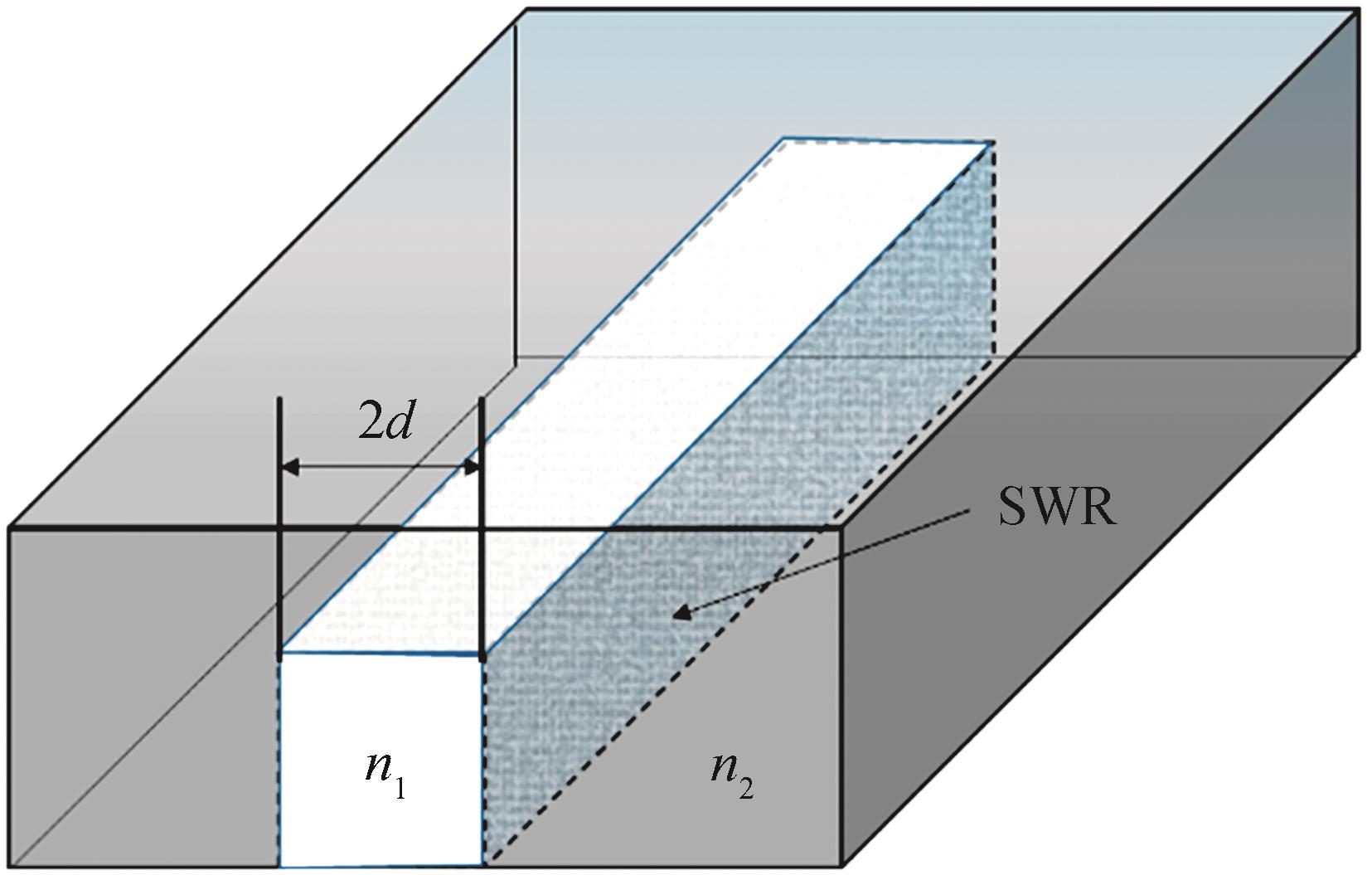
Set citation alerts for the article
Please enter your email address