
- Journal of Inorganic Materials
- Vol. 35, Issue 4, 491 (2020)
Abstract
As a new bismuth-based semiconductor material, BiOCl has recently become the focus of research in the field of photocatalysis and displays enhanced photocatalytic activity than TiO2 (P25, Degussa)[
Another effective method for enhancing photocatalytic performance is to modify the semiconductor with CQDs, which are attracting intense attention due to their environmentally friendly nature, chemical inertness, simple synthetic routes, ease of functionalization, high aqueous solubility, low cost and so on[
In a previous study, Xia, et al[
1 Experimental procedures
1.1 Preparation of CQDs/BiOCl nanocomposites
CQDs were prepared through a modified literature procedure[
In order to synthesize CQDs/BiOCl composites, 1 mmol of NaCl was dissolved into 17 mL of distilled water under stirring, then the solution was added into 17 mL of ethanol which contained 1 mmol of Bi(NO3)3·5H2O. After that, 0.5, 1.0, and 1.5 mL of CQDs solution (20 mg/mL) were dropped into the above mixture, respectively. The samples with 0.5, 1.0, and 1.5 mL of CQDs were denoted as C_0.5/BiOCl, C_1.0/BiOCl, and C_1.5/BiOCl, respectively. Correspondingly, the mass percentages of CQDs were calculated to be 3.7wt%, 7.1wt% and 10.3wt% for C_0.5/BiOCl, C_1.0/BiOCl, and C_1.5/BiOCl, respectively. Then the resulting suspension was transferred into an autoclave and kept at 100 ℃ for 18 h. After cooling to room temperature, the product was separated by centrifugation and washed with distilled water for several times, and then dried in air. For comparison, pure BiOCl was synthesized without the addition of CQDs.
1.2 Characterization
X-ray diffraction (XRD) patterns of the products were recorded on an X-ray diffractometer (Rigaku Co. Ltd., Tokyo, Japan) with Cu Kα radiation and in the 2θ range from 20° to 80°. An FEI tecnaiG2F30 transmission electron microscope (TEM) was used to observe the microstructure of the samples. UV-Vis diffuse reflectance spectra (DRS) of the products were obtained from a PE Lambda 900 UV-Vis spectrophotometer using BaSO4 as reference. The photocurrent measurements were conducted on a CHI 650 electrochemical workstation (Shanghai Chenhua, China) using a three-electrode system.
1.3 Photocatalytic test
Photocatalytic performances of the CQDs/BiOCl composites were tested by decomposition of RhB under a 500 W Xe lamp to simulate the solar light. During the experiment, 0.05 g of the photocatalyst was added to 50 mL of RhB (10-5 mol/L) solution to get a suspension, which was stirred magnetically in the dark for 1 h in order to establish an adsorption-desorption equilibrium between the pollutant molecules and the photocatalyst powders. 3 mL of suspension was collected at regular intervals, which was centrifuged to obtain a clarified solution for subsequent analysis. The concentration of RhB was analysed by recording the variations of the absorption band maximum (552 nm) on a UV-Vis spectrophotometer (754PC).
2 Results and discussion
2.1 Crystal structure
X-ray diffraction patterns of pure BiOCl and CQDs/BiOCl composites with different contents of CQDs are shown in Fig. 1. It can be seen that all the products are well crystallized, with all diffraction peaks consistent with the tetragonal BiOCl according to JCPDS 06-249. The introduction of CQDs has no obvious influence on the phase purity and crystallinity of the products. No diffraction peaks derived from CQDs can be observed, which is probably due to the low content and poor crystallinity of CQDs.
Figure 1.XRD patterns of the products
2.2 Microstructure
The microstructure of CQDs and the CQDs/BiOCl composite (C_1.0/BiOCl) were observed by the transmission electron microscope (TEM). Fig. 2(A) shows that the as-prepared CQDs are monodisperse with diameters of ca. 5 nm. Fig. 2(B, C) show that the CQDs/ BiOCl composite exhibits nanosheet structure of about 200 nm. HRTEM image is further used to investigate the fine structure of the CQDs/BiOCl composite (Fig. 2(D)). The lattice fringes at 0.335 nm in the HRTEM image agrees well with fringe spacing of (101) plane of BiOCl, and the lattice fringes at 0.321 nm coincide with (002) spacing of CQDs. These results further confirm that the CQDs/BiOCl nanocomposite has been successfully constructed with CQDs attached on the surface of BiOCl nanosheets.
Figure 2.TEM images of CQDs (A) and CQDs/BiOCl composite (B, C) and high resolution TEM image of CQDs/BiOCl composite (D)
2.3 UV-Vis diffuse reflectance spectra
UV-Visible diffuse absorption spectra (DRS) of pure BiOCl and CQDs/BiOCl composites are compared in Fig. 3. According to Fig. 3, no absorption in the visible region can be observed for pure BiOCl, and it only has photo-absorption at UV light region with absorption edge located at ca. 360 nm. However, when the BiOCl nanosheets are modified with CQDs, the absorption edge of CQDs/BiOCl composites are red-shifted to the visible range. Moreover, as the amount of CQDs increases, the absorption edge shifts monotonically to the longer wavelengths. This result indicates that CQDs play a crucial role in harvesting visible light, which implies that more efficient utilization of the sunlight can be realized.
Figure 3.UV-Vis diffuse reflectance spectra of pure BiOCl and CQDs/BiOCl composites
2.4 Photocatalytic activity
The photocatalytic activities of the as-prepared products are evaluated by degrading RhB under simulated sunlight irradiation. Fig. 4(A) shows the photo-degradation rates of RhB by pure BiOCl, CQDs and CQDs/BiOCl composites. With the CQDs amount increasing, the photo-degradation rate of RhB increases initially and achieves a maximum at CQDs amount of 1 mL. Complete degradation of RhB is realized by sample C_1.0/BiOCl within 2 min under simulated solar light irradiation, while the degradation rate of RhB is only 29.5% in the presence of pure BiOCl. However, further increasing the amount of CQDs leads to a decreased photocatalytic activity, which implies that there is an optimal loading amount of CQDs. This can be ascribed to that excess amount of CQDs will compete with BiOCl for light harvest, which decreases the absorption of light for degradation of RhB. Moreover, control experiment by using CQDs only under identical conditions is performed, and the result shows that the decolorization of RhB is negligible in the presence of CQDs alone. This result indicates that the decolorization is due to the photocatalysis but not the adsorption of dye molecules on the surface of CQDs.
Figure 4.(A) Photocatalytic degradation of RhB by CQDs, pure BiOCl and CQDs/BiOCl composites under simulated sunlight irradiation, and (B) stability test of the CQDs/BiOCl composite
Moreover, the cycling stability of the CQDs/BiOCl composite is tested through circulating runs. As shown in Fig. 4(B), the photocatalytic activity of the CQDs/BiOCl composite remains basically unchanged after being reused for five runs, which indicates that the CQDs/BiOCl composite has good photocatalytic stability and recyclability.
2.5 Mechanism of the enhanced photo-activity
It has been reported that the upconversion emission of CQDs can enhance the photocatalytic activities of CQDs modified composite photocatalysts under visible light irradiation[
Another crucial factor that determines the photocatalytic performance is the separation rate of the photo-induced charge carriers, which can be reflected directly by the photocurrent produced by the photocatalyst[
Figure 5.Photocurrent responses of BiOCl and CQDs/BiOCl composite
In order to further elucidate the photocatalytic mechanism, the main oxidative species are determined by radicals trapping experiments, using benzoquinone as superoxide radical (•O2-) scavenger, EDTA-2Na as holes scavenger and tert-butanol (t-BuOH) as hydroxyl radical (•OH) scavenger, respectively[
Figure 6.Trapping experiments of oxidative species during photo-degradation of RhB by CQDs/BiOCl composite
Based on the above experimental results, a mechanism is proposed to explain the enhanced photo-activity of the CQDs/BiOCl composite, as illustrated in Fig. 7. Under the irradiation of visible light, CQDs with upconversion luminescence behavior can convert the visible light into ultraviolet light, which can be absorbed by BiOCl to generate electron-hole pairs. On the other hand, CQDs as an excellent electron reservoir can trap electrons from the conduction band of BiOCl, and then transfer the electrons quickly to the surface of the photocatalyst. In this way, the charge recombination is restricted effectively, while the migration of the charge carriers is promoted significantly. The transferred electrons as good reducing agents can react with the adsorbed O2 on the surface of CQDs to yield superoxide radicals (•O2-), which are highly oxidative agents and can oxidize the pollutant molecules. Moreover, the long-lived holes on the valence band of BiOCl with strong oxidability can react with the RhB molecules directly, as confirmed by the radicals trapping experiments. Above all, due to the efficient harvest of sunlight, as well as the fast separation and migration of the photo-induced electron-hole pairs, the CQDs/BiOCl composite exhibits excellent photocatalytic performance, which can decolorize RhB in a short period of only 2 min.
Figure 7.Schematic illustration for the improved photo-activity of the CQDs/BiOCl composite
3 Conclusions
In summary, a highly efficient CQDs/BiOCl composite photocatalyst has been successfully prepared through a modified solvothermal process. Due to upconversion luminescence effect and photo-induced electron transfer ability of CQDs, the photo-absorption range of BiOCl is extended, while the recombination of photo-generated charge carriers is effectively suppressed. Consequently, the as-synthesized CQDs/BiOCl composite shows excellent photocatalytic performance under simulated solar light irradiation, which can decolorize RhB within only 2 min. This study demonstrates that introducing CQDs is an effective way to enhance the photocatalytic activity of the semiconductors.
References
[1] Y LEI, G WANG, S SONG et al. Synthesis, characterization and assembly of BiOCl nanostructure and their photocatalytic properties. CrystEngComm, 11, 1857-1862(2009).
[2] Q YE L, J DENG K, F XU et al. Increasing visible-light absorption for photocatalysis with black BiOCl. Physical Chemistry Chemical Physics, 14, 82-85(2012).
[3] X ZHANG, Z AI, F JIA et al. Generalized one-pot synthesis, characterization, and photocatalytic activity of hierarchical BiOX (X=Cl, Br, I) nanoplate microspheres. Journal of Physical Chemistyr C, 112, 747-753(2008).
[4] J HENLE, P SIMON, A FRENZEL et al. Nanosized BiOX (X=Cl, Br, I) particles synthesized in reverse microemulsions. Chemistry of Materials, 19, 366-373(2007).
[5] L ZHANG K, M LIU C, Q HUANG F et al. Study of the electronic structure and photocatalytic activity of the BiOCl photocatalyst. Applied Catalysis B-Environmental, 68, 125-129(2006).
[6] Y CHAI S, J KIM Y, H JUNG M et al. Heterojunctioned BiOCl/Bi2O3, a new visible light photocatalyst. Journal of Catalysis, 262, 144-149(2009).
[7] B LI T, G CHEN, C ZHOU et al. New photocatalyst BiOCl/BiOI composites with highly enhanced visible light photocatalytic performances. Dalton Transactions, 40, 6751-6758(2011).
[8] T LI H, D HE X, Y LIU et al. One-step ultrasonic synthesis of water-soluble carbon nanoparticles with excellent photoluminescent properties. Carbon, 49, 605-609(2011).
[9] B TANG L, B JI R, K CAO X et al. Deep ultraviolet photoluminescence of water-soluble self-passivated graphene quantum dots. ACS Nano, 6, 5102-5110(2012).
[10] C RAY S, A SAHA, R JANA N et al. Fluorescent carbon nanoparticles: synthesis, characterization, and bioimaging application. Journal of Physical Chemistry C, 113, 18546-18551(2009).
[11] J ZONG, H ZHU Y, L YANG X et al. Synthesis of photoluminescent carbogenic dots using mesoporous silica spheres as nanoreactors. Chemical Communications, 47, 764-766(2011).
[12] H SHEN J, H ZHU Y, L YANG X et al. One-pot hydrothermal synthesis of graphene quantum dots surface-passivated by polyethylene glycol and their photoelectric conversion under near-infrared light. New Journal of Chemistry, 36, 97-101(2012).
[13] Y LI, Y HU, Y ZHAO et al. An electrochemical avenue green- luminescent graphene quantum dots potential electron-acceptors photovoltaics. Advanced Materials, 23, 776-780(2011).
[14] W SHI, H LI X, M MA H. A tunable ratiometric pH sensor based on carbon nanodots for the quantitative measurement of the intracellular pH of whole cells. Angewandte Chemie International Edition, 51, 6432-6435(2012).
[15] N BAKER S, A BAKER G. Luminescent carbon nanodots: emergent nanolights. Angewandte Chemie International Edition, 49, 6726-6744(2010).
[16] C ZHANG H, H MING, Y LIAN S et al. Fe2O3/carbon quantum dots complex photocatalysts and their enhanced photocatalytic activity under visible light. Dalton Transactions, 40, 10822-10825(2011).
[17] T LI H, H LIU R, Y LIU et al. Carbon quantum dots/Cu2O composites with protruding nanostructures and their highly efficient (near) infrared photocatalytic behavior. Journal of Materials Chemistry, 22, 17470-17475(2012).
[18] H YU, C ZHANG H, H HUANG et al. ZnO/carbon quantum dots nanocomposites: one-step fabrication and superior photocatalytic ability for toxic gas degradation under visible light at room temperature. New Journal of Chemistry, 36, 1031-1035(2012).
[19] H ZHANG, H HUANG, H MING et al. Carbon quantum dots/Ag3PO4 complex photocatalysts with enhanced photocatalytic activity and stability under visible light. Journal of Materials Chemistry, 22, 10501-10506(2012).
[20] Y LIU J, Y LIU N, Z HAN Y et al. Metal-free efficient photocatalyst for stable visible water splitting
[21] J YU H, R SHI, F ZHAO Y et al. Smart utilization of carbon dots in semiconductor photocatalysis. Advanced Materials, 28, 9454-9477(2016).
[22] J KE, Y LI X, D ZHAO Q et al. Upconversion carbon quantum dots as visible light responsive component for efficient enhancement of photocatalytic performance. Journal of Colloid and Interface Science, 496, 425-433(2017).
[23] D HU Y, F XIE X, X WANG et al. Visible-light upconversion carbon quantum dots decorated TiO2 for the photodegradation of flowing gaseous acetaldehyde. Applied Surface Science, 440, 266-274(2018).
[24] J DI, X XIA J, X JI M et al. Carbon quantum dots modified BiOCl ultrathin nanosheets with enhanced molecular oxygen activation ability for broad spectrum photocatalytic properties and mechanism insight. ACS Applied Materials & Interfaces, 7, 20111-20123(2015).
[25] X GUO C, D ZHAO, Q ZHAO et al. Na+-functionalized carbon quantum dots: a new draw solute in forward osmosis for seawater desalination. Chemical Communications, 50, 7318-7321(2014).
[26] H LI, X HE, Z KANG et al. Water-soluble fluorescent carbon quantum dots and photocatalyst design. Angewandte Chemie International Edition, 49, 4430-4434(2010).
[27] X ZHANG, H HUANG, J LIU et al. Carbon quantum dots serving as spectral converters through broadband upconversion of near- infrared photons for photoelectrochemical hydrogen generation. Journal of Materials Chemistry A, 1, 11529-11533(2013).
[28] J ZHANG Z, T ZHENG T, Y XU J et al. Carbon quantum dots/Bi2WO6 composites for efficient photocatalytic pollutant degradation and hydrogen evolution. Nano, 12, 1750082(2017).
[29] G KIM H, H BORSE P, Y CHOI W et al. Photocatalytic nanodiodes for visible light photocatalysis. Angewandte Chemie International Edition, 44, 4585-4589(2005).
[30] Y ZHU Y, F LIU Y, H LV Y et al. Enhancement of photocatalytic activity for BiPO4
[31] D YUE, M CHEN D, H WANG Z et al. Enhancement of visible photocatalytic performances of a Bi2MoO6-BiOCl nanocomposite with plate-on-plate heterojunction structure. Physical Chemistry Chemical Physics, 16, 26314-26321(2014).
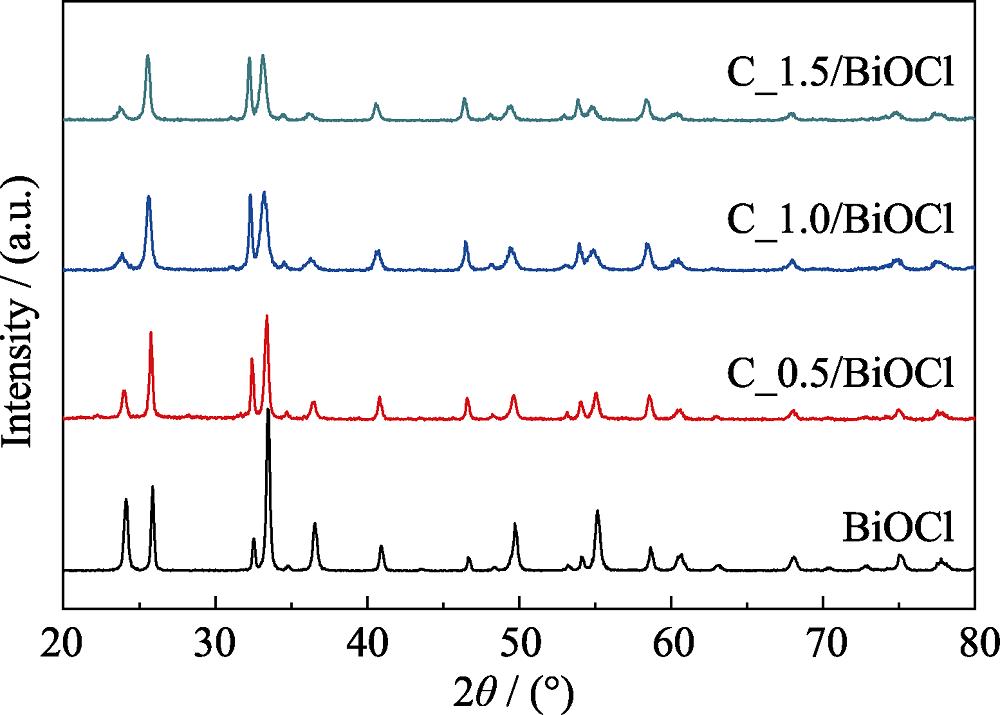
Set citation alerts for the article
Please enter your email address