Abstract
Dysprosium-doped orthorhombic yttrium aluminate ( or Dy:YAP) single crystals were grown by the Czochralski method with a size of . Based on the measurements of spectra and theoretical analysis, the white-light emission was investigated with different doping concentrations. The optimal white emission was achieved at doping concentration of 1.0% under 450 nm excitation. Combining with residual pumping light, the white-light output was successfully obtained with Commission Internationale de l´Eclairage (CIE) coordinates x, y, the color temperature of 4000 K, and the largest fluorescence quantum yield of 46.9%. With the development of the GaN laser diode, the Dy:YAP single crystal has proven applicable in white-light-emitting diodes.1. Introduction
Recently, white-light-emitting diodes (W-LEDs) have attracted considerable interest because of their outstanding advantages, such as high brightness, low energy consumption, a long lifetime span, and environmental friendliness[1–6]. Presently, commercial W-LEDs are achieved by pumping () phosphor using GaN chips or three primary colors of emitting phosphors using InGaN chips[7]. However, there are certain deficiencies of this type, for instance, poor heat resistance of the epoxy resins, a light scattering of inhomogeneous phosphors, and chromatic aberration after a long period of operation[8]. Therefore, looking for a matrix material with good white-light properties has become a research hotspot. In order to offset these drawbacks, much attention has been applied to single-host emitting glasses, glass ceramics, transparent ceramics, and single crystals, based on borates containing light activators, such as , , , , , and [9,10]. Compared with glasses and ceramics, LED applications based on single crystals tend to obtain high stability, a long lifetime, and high luminous efficiency because of the crystals’ excellent physical and chemical properties.
Dysprosium ion () is one of the typical rare-earth ions. contains multiple energy level transitions at visible emission bands, such as (645–665 nm), (570–600 nm), and (470–500 nm)[11,12], as shown in Fig. 1. The strongest fluorescence emission is at the yellow band (573 nm), and the emission is weak at the blue and red bands, which causes the emitted fluorescence to tend to be yellow light. In addition to ultraviolet absorption, it also has an absorption at the blue band (450 nm). Using color compensation from the remaining pump blue, the emission of ions can be achieved in the warm white light. There have been many reports on the white-light luminescence of rare-earth-doped crystals, such as [13], [9], [14], [15], and [16], which indicate that ions or co-doped single crystals have the potential to emit white light when excited by ultraviolet (UV) or blue light.
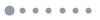
Figure 1.Energy-level diagram of Dy3+ systems.
Compared with fluoride crystals and phosphate crystals, the orthorhombic yttrium aluminate crystal (YAP) has a larger emission cross section, better thermal properties, a higher damage threshold, and better mechanical properties[17–20], which leads rare ion-doped YAP crystals to have better luminescence or laser performances. However, its anisotropy makes it difficult to obtain high-quality and large-scale single crystals, which is the main barrier to widespread applications. They are vulnerable to cracking and disintegration during the cooling down stage of crystal growth because the thermal expansion coefficients of YAP crystals differ significantly in different crystallographic axes, and the lattice constants described by the Pnma space group are very similar between and , while they differ significantly for [21].
Sign up for Chinese Optics Letters TOC. Get the latest issue of Chinese Optics Letters delivered right to you!Sign up now
In this paper, an effective white-light-emitting option was demonstrated with the Dy:YAP single crystal excited by UV or blue light. Based on theoretical analysis and performance characterization, the doping concentration of Dy:YAP crystals was optimized to be 1% (atomic fraction) for better luminous performance. With an excitation wavelength of 450 nm, white-light experiments were performed. Due to the blending of pumping blue light, the obtained white-light was calculated with CIE coordinates , , and the color temperature changed from 3700 to 4000 K. This indicates that the -doped YAP single crystal has a potential application in W-LEDs excited by blue light.
2. Experiment
Crystal growth. YAP crystals were grown by the Czochralski method, using high-purity and . An iridium crucible with a diameter of 120 mm was used as the container and heat generator. High-purity argon was adopted as the shielding gas atmosphere. The axis YAP crystals were used as seed crystals, and the growth rate was 1–2 mm/h at 10–15 r/min. By altering the upper insulation cavity’s structural dimensions in the temperature field, such as by reducing the upper insulation cavity’s inner diameter, the upper insulation cover’s opening diameter, and the height of the upper insulation cavity, it is possible to prevent the crystals from being exposed to the protective gas with a significant temperature difference from the environment because of the protective gas’s exit cavity. After the crystal growth processes of seeding, shouldering, equilibration, and tailing, the Dy:YAP crystals were grown by lowering the temperature to room temperature after 20–40 h at different cooling rates.
Structural characterization. X-ray diffraction (XRD) analysis was implemented at room temperature on a Bruker D2 PHASER diffractometer equipped with Cu Kα radiation. The range is 10°–80° with a step of 0.02° and a counting time of 1 s/step. Single-crystal X-ray diffraction data were also collected on a Bruker SMART APEX II 4 K CCD diffractometer equipped with Mo Kα radiation. The structure was handled using the direct technique of SHElXS-201443. All the atoms in the structure were then refined using the full-matrix least-squares method. When the structure’s symmetry was checked using PLATON, no greater symmetry was discovered.
Optical spectral property measurements. The absorption spectra were recorded with a dimension of crystal along the , , cut on a UV–VIS–NIR spectrophotometer (UH4150, Hitachi) wavelength range between 190 and 2000 nm. The excitation spectra, fluorescence lifetime, photoluminescence (PL) spectra, and fluorescence quantum yield were measured by a fluorescence spectrum analyzer (Edinburgh FLS-980).
White-light property measurements. The white light was pumped by a 450 nm GaN laser diode (LD) with a spot diameter of 200 µm. The dimensions of the Dy:YAP sample were . The whole system contains only a blue LD pump, a laser alignment system, and a Dy:YAP crystal. In addition, the spectrum of the white light generated by the Dy:YAP was measured by an optical spectrum analyzer (Advantest Q8381A).
3. Results and Discussion
The grown 1% Dy:YAP crystal and test samples are shown in Fig. 2(a) with the crystal dimensions of and processed sample sizes of and for further measurements and white-light emission experiments. From Fig. 2(a), the obtained crystal has no cracks and exhibits good quality. XRD patterns and standard ICSD 4115 are shown in Fig. 2(b). As can be seen, the patterns are nearly identical because the doping concentration of is relatively low, and the radii of and are comparable. It is hard to observe the structure differences using X-ray single-crystal diffraction or X-ray powder diffraction.
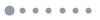
Figure 2.(a) Photographs and test samples of Dy:YAP crystal with a doping concentration of 1%; (b) XRD patterns of 1% Dy:YAP crystal and standard ICSD 4115.
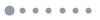
Figure 3.Crystal structure of YAP crystal.
The structure of the Dy:YAP single-crystal sample was measured using an X-ray single-crystal diffractometer. The results are shown in Fig. 3. Because the diameters of and ions are comparable, there is barely a difference. YAP crystals belong to the orthorhombic crystal system with space group Pnma and negative biaxial crystals with two optical axes at an angle of 70° in the plane and the axis as an acute isometry[20]. The oxygen coordination around the ion is approximately cubic, but there are distortions in the and polyhedra, so the YAP crystal structure is a distorted chalcogenide structure[21]. There are four molecules in the unit cell of the YAP crystal, and the coordination number of is 6, which is in the center of the oxygen octahedron, while the coordination number of is 12, which is in the center of the oxygen coordination polyhedron. The lattice constants are , , and . The bonds length of Al-O, Y-Al, and Y-O are 1.84 Å, 3.18 Å, and 2.62 Å, respectively. The Y-O bond is longer than the Y-O bond of the YAG crystal (2.45 Å), which is more favorable for the entry of rare-earth ions to replace ions[22].
The absorption spectra (absorbance or Abs) of Dy:YAP single crystals were recorded with a spectrophotometer in the 300–2000 nm wavelength range and are shown in Fig. 4(a). The Dy:YAP crystal absorption peak is located at 350 nm along the -cut. In order to accurately determine the absorption band from 300 to 500 nm, the excitation spectra were measured by a fluorescence spectrum analyzer (Edinburgh FLS-980), as shown in the inset of Fig. 4(b). From this, the Dy:YAP crystal’s maximum absorption peak is located at 350 nm. Absorption bands center around 325, 350, 365, 388, 427, 450, and 475 nm, which are relevant to transitions from the ground state to excited states , , , , , , and , respectively. It also has an absorption peak at 450 nm, which shows that commercial GaN LDs can effectively stimulate the crystal to generate red and yellow light[23]. Combined with input blue light, the Dy:YAP crystal could be a candidate for white-light application. For the purpose of optimizing the doping concentration, Figs. 4(c) and 4(d) display the measurements of the Dy:YAP crystal’s PL spectra at excitation wavelengths of 350 and 450 nm, respectively. It is evident from Figs. 4(c) and 4(d) that the emission spectra of the crystal exhibit a blue band at 483 nm () and a yellow band at 573 nm (), when excited by pumping resources. It should be noted that emission intensities at 483 and 573 nm under excitation at 450 nm are weaker than that at 350 nm. These findings demonstrate that a Dy:YAP crystal may produce both blue and yellow light when properly stimulated by UV light. With the development of a blue LD, Dy:YAP crystals are also suitable for the packaging of the application with 450 nm stimulation for economic consideration because of their relatively moderate intensity. From Fig. 4’s PL spectra of the Dy:YAP crystal, the emission intensity will increase first and then decrease with the increasing doping concentration. The critical doping concentration was around 1%.
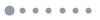
Figure 4.(a) Absorption spectra of Dy:YAP single crystals; (b) excitation spectra of Dy:YAP crystals with different doping concentrations; (c) PL spectra of Dy:YAP crystals with different doping concentrations and excited at 350 nm; (d) PL spectra of Dy:YAP crystals with different doping concentrations and excited at 450 nm.
According to the above spectra, the CIE chromaticity coordinates of the Dy:YAP crystal can be calculated with different exciting wavelengths; the results are shown in Fig. 5. With the exciting wavelength of 350 nm, the CIE chromaticity coordinates are , , and the color temperature is 3700 K. The chromaticity coordinates will move away from the white light with increasing doping concentrations. Figure 5(b) shows the CIE chromaticity coordinates of Dy:YAP with the exciting wavelength of 450 nm, the coordinates , , and the color temperature . As demonstrated in Fig. 5, the calculated coordinates lean toward the yellow zone, and the temperature colors deviate from the standard white to warm white. Considering that pump light can also be involved in chromaticity compensation, GaN LD with 450 nm excitation is both suitable for pumping () and reconcilable for color temperature. After thorough consideration, 1% doping concentration -cut samples were selected for the white-light experiment with GaN LD pumping.
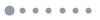
Figure 5.CIE chromaticity coordinates of Dy:YAP crystals with different exciting wavelengths and doping concentrations.
The white-light experiment was set up following Fig. 6(a). Dy:YAP was pumped using 450 nm generated by a GaN LD. The optical power density of the adopted blue LD is with a spot diameter of 200 µm. The whole system contains only a blue LD pump, a laser alignment system, and a Dy:YAP crystal with dimensions of [inset, Fig. 2(a)], which ensures that the pass length is long enough to fully absorb the pump blue light. The white-light system output from Dy:YAP could be extremely simplified, which, on the other hand, indicates that the application of Dy:YAP for industrial purposes can greatly reduce expenses. It can be observed that the whole crystal sample is emitting bright shining white radiation.
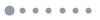
Figure 6.(a) Schematic of the Dy:YAP white light; (b) white-light spectrum; (c) CIE chromaticity coordinates.
The white-light spectrum was collected by an optical spectrum analyzer (Advantest Q8381A) on the side of the crystal, as shown in Fig. 6(b). As can be seen, the emission spectrum contains 573 nm (), 483 nm (), and a residual blue band. The CIE chromaticity coordinates of the Dy:YAP crystal calculated based on the corresponding emission spectrum are summarized in Fig. 6(c). The color temperature is 4000 K for coordinates , . Compared with theoretical calculations, the output white light tends to be warm light with compensation for the blue light. Since the compensation is only from the residual incident blue light, the color temperature should rise as the length of the crystal samples increases. Relative experiments will be carried out in the future.
Fluorescence quantum yield (PLQY) of Dy:YAP crystals with different doping concentrations are recorded in Fig. 7. The maximum value is 46.9% and 26.7% when excited by 350 and 450 nm. The fitted curve of PLQY to the concentration of is also simulated in Fig. 7(c). As can be seen, the PLQY reaches its highest point at 1% and decreases rapidly as the concentration of increases. This phenomenon is due to cross-relaxation mechanisms within the system of ions during concentration quenching. The long lifetime of the lower-energy level of the ion tends to lead to particle number blockage, which in turn affects the laser effect. With the increase in doping concentration, laser output would exhibit a dramatic decrease.
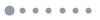
Figure 7.(a) PLQY with the excited wavelength of 350 nm; (b) PLQY with the excited wavelength of 450 nm; (c) PLQY with different doping concentrations; (d) luminescent lifetime of 1% Dy:YAP crystal.
Compared with other crystals doped with , listed in Table 1, Dy:YAP crystals show a higher quantum efficiency of 46.9%, slightly higher than the 39.2% of Dy:YAG[5]. Different quantum efficiencies of ions vary in different crystals, which is determined by the host crystals’ structure. YAP crystals have the structure of a distorted perovskite, and the disorder of the crystal structure results in broad absorption and emission spectra similar to glasses. At the same time, it still possesses excellent thermal properties. Combining their outstanding physical and chemical properties, Dy:YAP crystals have an incomparable future for white-light applications. Figure 7(d) describes the results of a luminescent lifetime measurement on a 1% Dy:YAP crystal at 573 nm. The excitation and monitoring wavelengths are 350 and 573 nm, respectively. As shown in Fig. 7, the 1% sample exhibits a single exponential decay behavior with a lifetime of 0.54 ms.
Crystals | Efficiency (%) |
---|
Dy:YAP | 46.9 |
Dy:YAG[7] | 39.2 |
[22] | 7 |
[23] | 21.7 |
Dy, [24] | 12.88 |
[25] | 25 |
[26] | 15.16 |
[11] | 16.6 |
Table 1. Fluorescence Quantum Efficiency of Various Dy3+-Doped Crystals
4. Conclusion
In summary, we demonstrated an effective white LED with the Dy:YAP single crystal excited by UV or blue light. Based on theoretical analysis and performance characterization, the optimal doping concentration 1% Dy:YAP crystal has the better luminous performance with a luminescent lifetime of 0.54 ms and a PLQY of 46.9%. White-light emission was achieved under excitation by a commercialized blue LD (450 nm), with CIE coordinates , , the color temperature , and a PLQY of 26.7%, which forecasts that the -doped YAP single crystal has potential applications in W-LEDs, especially suitable for the development tendency of GaN LDs.
References
[1] X. Li, S. Zhou, R. Wei, X. Liu, B. Cao, H. Guo. Blue–green color-tunable emissions in novel transparent Sr2LuF7:Eu/Tb glass-ceramics for WLEDs. Chin. Opt. Lett., 18, 051601(2020).
[2] Y. Hao, Y. Yao, H. Shi, H. Yu, Y. Jiang, L. Ma. Frequency control of a lattice laser at 759 nm by referencing to Yb clock transition at 578 nm. Chin. Opt. Lett., 20, 120201(2022).
[3] Y. Zhang, J. Zou, W. Zheng, K. Feng, B. Xu, Z. Yu. Watt-level continuous-wave intracavity frequency-doubled Pr:YLF-LBO laser at 320 nm. Chin. Opt. Lett., 19, 091406(2021).
[4] H. Su, H. Zhang, W. Wu, X. Wang, G. Wang, L. Zhou. Chemically engineered dendrite growth of uniform monolayers MoS2 for enhanced photoluminescence [Invited]. Chin. Opt. Lett., 20, 011602(2022).
[5] M. Jin, Z. Qian, X. Chen, X. Cui, K. Jiang, X. Sun, D. Li, P. Tian. Signal transmission of 4 GHz beyond the system bandwidth in UV-C LED communication based on temporal ghost imaging. Chin. Opt. Lett., 19, 110602(2021).
[6] H. Yu, L. Su, X. Qian, D. Jiang, Q. Wu, F. Tang, J. Wang, J. Xu. Influence of Gd3+ on the optical properties of Dy3+-activated CaF2 single crystal for white LED application. J. Electron. Mater., 48, 2910(2019).
[7] Y. Pan, S. Zhou, D. Li, B. Liu, Q. Song, J. Liu, P. Liu, Y. Ding, X. Wang, X. Xu, J. Xu. Growth and optical properties of Dy:Y3Al5O12 crystal. Physica B, 530, 317(2018).
[8] S. Yu, Q. H. Zhang, H. C. Hu, Z. L. Chen, D. C. Liu, Y. N. Liang, F. P. Liang. Tuning slow magnetic relaxation behaviour in a {Dy2}-based one-dimensional chain via crystal field perturbation. RSC Adv., 10, 11831(2020).
[9] L. Fang, X. Zhou, J. Zhang, H. Xia, B. Chen, H. Song. Control of white light emission via co-doping of Dy3+ and Tb3+ ions in LiLuF4 single crystals under UV excitation. J. Mater. Sci.: Mater. Electron., 31, 3405(2020).
[10] D. Jiang, Y. Jiang, H. Xia, J. Zhang, S. Yang, X. Gu, H. Jiang, B. Chen. Growth and optical properties of Dy3+/Eu3+ co-doped NaYF4 single crystals with cubic lattice for white LED application. Opto-Electron. Lett., 11, 356(2015).
[11] J. Long, F. Chu, Y. Wang, C. Zhao, W. Dong, X. Yuan, C. Ma, Z. Wen, R. Ma, M. Du, Y. Cao. M8MgSc(PO4)7:xDy3+ (M = Ca/Sr) single-phase full-color phosphor with high thermal emission stability. Inorg. Chem., 56, 10381(2017).
[12] Y. Liu, B. Lei, C. Shi. Luminescent properties of a white afterglow phosphor CdSiO3:Dy3+. Chem. Mater., 17, 2108(2004).
[13] Y. Dong, H. Xia, L. Fu, S. Li, X. Gu, J. Zhang, D. Wang, Y. Zhang, H. Jiang, B. Chen. White light emission from Dy3+-doped LiLuF4 single crystal grown by Bridgman method. Opto-Electron. Lett., 10, 262(2014).
[14] Y. Jiang, H. Xia, J. Zhang, S. Yang, H. Jiang, B. Chen. Growth and optical spectra of Tb3+/Eu3+ co-doped cubic NaYF4 single crystal for white light emitting diode. J. Mater. Sci. Technol., 31, 1232(2015).
[15] Z. Zhang, Y. Zhang, Z. Feng, W. Cheng, H. Xia, X. Zhang. Luminescent properties of Ce3+/Tb3+ co-doped glass ceramics containing YPO4 nanocrystals for W-LEDs. J. Rare Earth, 34, 464(2016).
[16] F. Xu, X. Zhou, H. Xia, H. Song, B. Chen. Highly thermally stable Dy3+/Sm3+ co-doped Na5Y9F32 single crystals for warm white LED. J. Phys. Chem. Solids, 158, 110240(2021).
[17] M. J. Weber, M. Bass, K. Andringa, R. R. Monchamp, E. Comperchio. Czochralski growth and properties of YAlO3 laser crystals. Appl. Phys. Lett., 15, 342(1969).
[18] M. Weber, M. Bass, E. Comperchio, L. Riseberg. Ho3+ laser action in YAlO3 at 2.119 µm. IEEE J. Quantum. Electron., 7, 497(1971).
[19] M. J. Weber. Multiphonon relaxation of rare-earth ions in yttrium orthoaluminate. Phys. Rev. B, 8, 54(1973).
[20] K. L. Hovhannesyan, M. V. Derdzyan, A. V. Yeganyan, V. E. Kisel, A. S. Rudenkov, N. V. Kuleshov, A. G. Petrosyan. Single crystals of YAP:Yb for ultra short pulse lasers. J. Contemp. Phys. Arme., 55, 131(2020).
[21] B. Cockayne, B. Lent, J. S. Abell, I. R. Harris. Cracking in yttrium orthoaluminate single crystals. J. Mater. Sci., 8, 871(1973).
[22] N. S. Singh, R. S. Ningthoujam, M. N. Luwang, S. D. Singh, R. K. Vatsa. Luminescence, lifetime and quantum yield studies of YVO4:Ln3+ (Ln3+ = Dy3+, Eu3+) nanoparticles: concentration and annealing effects. Chem. Phys. Lett., 480, 237(2009).
[23] S. Huang, T. Feng, B. Jiang, M. Feng, Z. Shen, W. Wang, L. Zhang. J-O study of a novel Dy-doped Lu3Al5O12 transparent ceramic for potential application in yellow laser generation. J. Lumin., 231, 117763(2021).
[24] K. Mondal, J. Manam. Investigation of photoluminescence properties, thermal stability, energy transfer mechanisms and quantum efficiency of Ca2ZnSi2O7:Dy3+, Eu3+ phosphors. J. Lumin., 195, 259(2018).
[25] A. R. Beck, S. Das, J. Manam. Temperature dependent photoluminescence of Dy3+ doped LiCaBO3 phosphor. J. Mater. Sci.: Mater. Electron., 28, 17168(2017).
[26] X. Zhang, Z. Lu, F. Meng, L. Hu, X. Xu, J. Lin, C. Tang. Luminescence properties of Ca3Si2O7:Dy3+ phosphor for white light-emitting diodes. Mater. Lett., 79, 292(2012).