
- Chinese Optics Letters
- Vol. 20, Issue 6, 063701 (2022)
Abstract
Keywords
1. Introduction
Terahertz (THz) spectroscopy has made great progress in numerous fields owing to its attractive and exclusive attributes[
Geometry algebra (GA)[
The exterior product, an indispensable component of the geometric product, is involved in some applications. Li et al.[
Sign up for Chinese Optics Letters TOC. Get the latest issue of Chinese Optics Letters delivered right to you!Sign up now
In this study, we investigate the characteristic of exterior product constructed by the vectorized refractive index and absorption coefficient as a function of frequency and the further relationship between CMSE and absorption coefficient, with the refractive index resorting to the exterior product by reusing the experiment data in the previous work[
2. Method
It is acknowledged that cross product between vectors is restricted to operate in three dimensions in linear algebra[
3. Results and Discussion
The bandwidth for absorption coefficients and refractive indices of six concentrations of bovine serum albumin (BSA) solutions in Ref. [9] is from 0.1 to 0.5 THz with the resolution of 0.01 THz. From Eq. (3), taking 5000 µg/mL as an example, the mean profile of
Figure 1.Mean profile of
Figure 2.Linear fitting between A0 and modulus of the (a) absorption coefficient and (b) refractive index.
In order to further explore the relationship between CMSE and the refractive index or absorption coefficient in the framework of exterior product, similar to the bivector
In compliance with the method of our previous work, VMD was performed for that relationship investigation. In terms of the previous search results[
Figure 3.Distribution on standard deviation of CMSE over 20 scales at α = 210.
Figure 4.Linear fitting between B0 at (a) α = 200 and (b) α = 210 from scales 15 to 20 and A0 from 0.2 to 0.38 THz.
Figure 5.Linear fitting between B0 from scales 15 to 20 and modulus of (a) absorption coefficient and (b) refractive index from 0.2 to 0.38 THz.
Additionally, the correlation on the coefficients of bivectors between
Figure 6.Profile of the coefficients of (a)
Figure 7.Linear fitting between coefficients of
Figure 8.Weight distribution on coefficients of bivector (a)
4. Conclusions
In this work, by reanalyzing the data of previous work, we further study the relationship between CMSE and refractive index or absorption coefficient under the framework of exterior product, which presents a novel insight into the relationship between THz time and frequency domain information. The quantity bivectors
References
[1] Y. Peng, C. Shi, X. Wu, Y. Zhu, S. Zhuang. Terahertz imaging and spectroscopy in cancer diagnostics: a technical review. BME Frontiers, 2020, 2547609(2020).
[2] P. Bawuah, J. A. Zeitler. Advances in terahertz time-domain spectroscopy of pharmaceutical solids: a review. TrAC Trends Anal. Chem., 139, 116272(2021).
[3] L. Afsah-Hejri, E. Akbari, A. Toudeshki, T. Homayouni, A. Alizadeh, R. Ehsani. Terahertz spectroscopy and imaging: a review on agricultural applications. Comput. Electron. Agric., 177, 105628(2020).
[4] L. Yang, T. Guo, X. Zhang, S. Cao, X. Ding. Toxic chemical compound detection by terahertz spectroscopy: a review. Rev. Anal. Chem., 37, 21(2018).
[5] S. Zhong. Progress in terahertz nondestructive testing: a review. Front. Mech. Eng., 14, 273(2019).
[6] S.-D. Wu, C.-W. Wu, S.-G. Lin, C.-C. Wang, K.-Y. Lee. Time series analysis using composite multiscale entropy. Entropy, 15, 1069(2013).
[7] R. Zhang, Y. He, K. Liu, L. Zhang, S. Zhang, E. P. MacPherson, Y. Zhao, C. Zhang. Composite multiscale entropy analysis of reflective terahertz signals for biological tissues. Opt. Express, 25, 23669(2017).
[8] H. Liu, K. Zhao, X. Liu, Z. Zhang, J. Qian, C. Zhang, M. Liang. Diagnosis of hepatocellular carcinoma based on a terahertz signal and VMD-CWSE. Biomed. Opt. Express, 11, 5045(2020).
[9] H. Liu, X. Liu, Z. Zhang, C. Zhang. Investigating the relationship between composite multiscale entropy and optical property of bovine serum albumin solutions using THz pulsed system. Opt. Lasers Eng., 137, 106374(2021).
[10] D. Hestenes, G. Sobczyk. Clifford Algebra to Geometric Calculus: A Unified Language for Mathematics and Physics(1984).
[11] R. Wang, M. Shen, T. Wang, W. Cao. L1-norm minimization for multi-dimensional signals based on geometric algebra. Adv. Appl. Clifford Algebras, 29, 33(2019).
[12] Y. Li, W. Liu, X. Li, Q. Huang, X. Li. GA-SIFT: a new scale invariant 500 feature transform for multispectral image using geometric algebra. Inf. Sci., 281, 559(2014).
[13] B. Jancewicz. Multivectors and Clifford Algebra in Electrodynamics(1989).
[14] D. Hestenes. Clifford algebra and the interpretation of quantum mechanics. Clifford Algehras and Their Applications in Mathematical Physics, 321(1986).
[15] F. J. Chinea. A Clifford algebra approach to general relativity. Gen. Relat. Gravit., 21, 21(1989).
[16] A. Lasenby, C. Doran, S. Gull. Gravity, gauge theories and geometric algebra. Philos. Trans. R. Soc. A, 356, 487(1998).
[17] W. Xie, J. Li, J. Pei. THz-TDS signal analysis and substance identification. J. Phys, 276, 012227(2011).
[18] W. Xie, J. Li, J. Pei. An analysis of THz-TDS signals using geometric algebra. Proc. SPIE, 7277, 72770E(2008).
[19] W. Xie, J. Li, J. Pei. THz-TDS signal analysis and substance identification via the conformal split. Sci. China Inf. Sci., 55, 49(2012).
[20] S. Zhou, D. G. Valchev, A. Dinovitser, J. M. Chappell, A. Iqbal, B. W.-H. Ng, T. W. Kee, D. Abbott. Terahertz signal classification based on geometric algebra. IEEE Trans. THz Sci. Technol., 6, 793(2016).
[21] Y. Xu, W. Hong, Z. Gao, C. Zheng. Geometric algebra representation principle of multivariate data dimension-increasing transformation. J. Yanshan Univ., 32, 393(2008).
[22] Y. Xu, W. Hong, Z. Gao. Geometric algebra multi-vector representation method of pattern features. J. Yanshan Univ., 34, 119(2010).
[23] J. Li, W. Hong. Feature extraction for breast cancer data based on geometric algebra theory and feature selection using differential evolution. J. Biomed. Eng., 31, 1218(2014).
[24] J. Li, W. Xie, J. Pei. Similarity metrics and their applications of THz-TDS signals. Signal Process., 27, 1457(2011).
[25] B. Mishra, M. Kochery, P. Wilson, R. Wilcock. A novel signal processing coprocessor for n-dimensional geometric algebra applications. Circuit. Syst., 5, 274(2014).
[26] L. Zou, J. Lasenby, Q. Wan, Z. He, M. Lv. Geometric algebra in electronics and information engineering: an introduction. Int. J. Elec. Eng. Educ., 53, 252(2016).
[27] M. El Baz, Y. Hassouni. Deformed exterior algebra, quons and their coherent states. Int. J. Mod. Phys. A, 18, 3015(2003).
[28] A. Singleton. Superconformal quantum mechanics and the exterior algebra. J. High Energ. Phys., 2014, 131(2014).
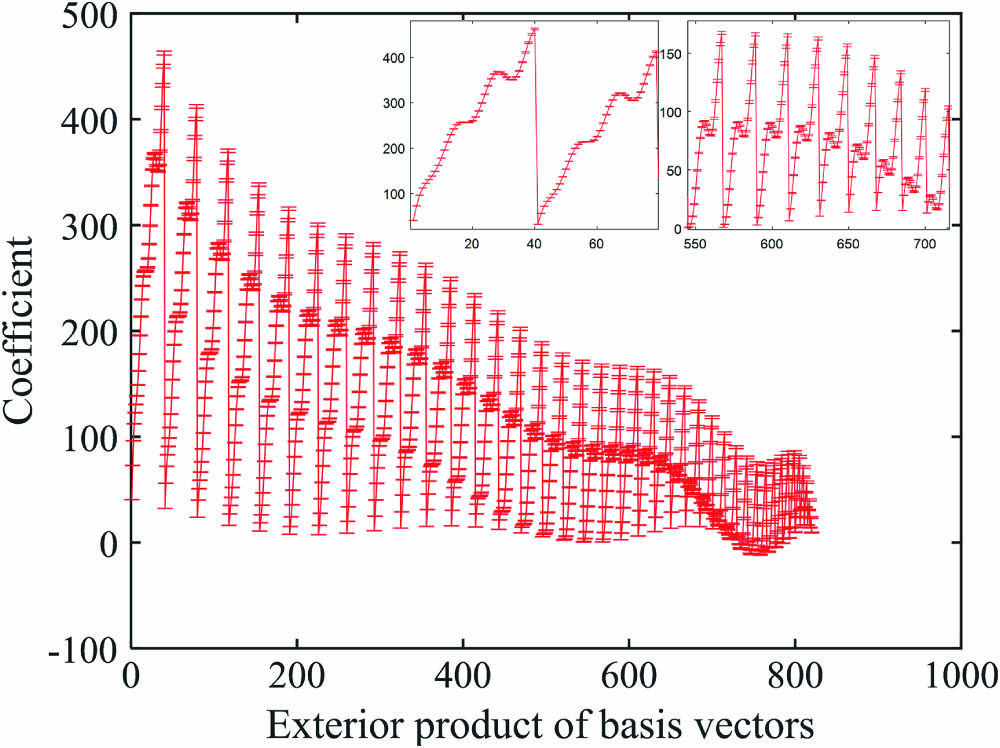
Set citation alerts for the article
Please enter your email address