
- Chinese Optics Letters
- Vol. 20, Issue 11, 111402 (2022)
Abstract
Keywords
1. Introduction
A
Generally,
Sign up for Chinese Optics Letters TOC. Get the latest issue of Chinese Optics Letters delivered right to you!Sign up now
In this Letter, a highly efficient AO
2. Experimental Setup
The schematic diagram of the AO
Figure 1.Schematic diagram of AO Q-switched Tm,La:CaF2 laser. AOM, acousto-optic modulator.
3. Results and Discussions
Firstly, the output power performance of the CW
Figure 2.Output power of CW and AO Q-switched laser. Insert, far-field three-dimensional intensity distribution of AO Q-switched laser at the maximum output power.
It is worth noting that the average output power as well as the slope efficiency of AO
The laser spectra were observed continuously from low power onwards as the pumping power increased. The spectral analyzer (MS3504i, made in Belarus) used in this work has a resolution of 0.3 nm. Figure 3 shows the CW laser spectrum and the AO
Figure 3.CW and Q-switched laser emission spectra.
The pulse laser performance was recorded in detail. Figure 4 shows the relationship between pulse durations of AO
Figure 4.Curves of pulse width versus absorbed pump power.
The single pulse energy and peak power of the AO
Figure 5.Curves of single pulse energy versus absorbed pump power.
Figure 6.Curves of peak power versus absorbed pump power.
The AO actively
Figure 7.Temporal traces of Q-switched laser at time scales of 100 ns/div and 10 ms/div.
Table 1 summarizes the output characteristics of the AO
Crystal | Ref. | |||||
---|---|---|---|---|---|---|
1912 | 48 | 0.4 | 8.3 | – | [ | |
Tm:YAG | 2013 | 54 | 0.003 | 0.055 | – | [ |
Tm:LSO | 2040.1 | 345 | 0.26 | 0.75 | 12.3 | [ |
Tm:SSO | 1968 | 308 | 0.128 | 0.416 | – | [ |
1912 | 280 | 0.335 | 1.19 | 18.1 | [ | |
Tm:YAP | 1988 | 38 | 16.36 | 430 | 29.4 | [ |
Tm:YLF | 1879 | 37 | 1.97 | 53.2 | 36 | [ |
1881.7 + 1888.5 | 100 | 1.89 | 18.9 | 64.7 | Our work |
Table 1. Summaries of AO
Furthermore, milli-joule-level pulse energy of 1.89 mJ is achieved at the absorbed pump power of 2.02 W in our work and comparable to 1.97 mJ obtained from the Tm-doped yttrium lithium fluoride (Tm:YLF) laser at the absorbed pump power of 9.2 W[
4. Conclusions
In conclusion, a highly efficient dual-wavelength actively
References
[1] K. Scholle, S. Lamrini, P. Koopmann, P. Fuhrberg. 2 µm laser sources and their possible applications. Frontiers in Guided Wave Optics and Optoelectronics, 471(2010).
[2] A. Godard. Infrared (2–12 µm) solid-state laser sources: a review. C. R. Phys., 8, 1100(2007).
[3] L. Zhang, S. Sun, Z. Lin, H. Lin, G. Zhang, X. Mateos, J. M. Serres, M. Aguiló, F. Díaz, P. Loiko, Y. Wang, U. Griebner, V. Petrov, E. Vilejshikova, W. Chen. Crystal growth, spectroscopy and first laser operation of a novel disordered tetragonal Tm:Na2La4(WO4)7 tungstate crystal. J. Lumin., 203, 676(2018).
[4] I. T. Sorokina, K. L. Vodopyanov. Solid-State Mid-Infrared Laser Sources(2003).
[5] P. Liu, L. Jin, X. Liu, H. Haitao, J. Zhang, D. Tang, D. Shen. A diode-pumped dual-wavelength Tm, Ho:YAG ceramic laser. IEEE Photon. J., 8, 1504007(2016).
[6] X. Y. Liu, X. W. Li, S. Z. Zhao, K. J. Yang, L. Guo, T. Li, W. C. Qiao, M. Li, B. T. Zhang, J. L. He, L. H. Zheng, L. B. Su, J. Xu. Dual-wavelength synchronously mode-locked Tm-doped bulk laser with terahertz frequency beating. Chin. Opt. Lett., 17, 091401(2019).
[7] L. C. Kong, Z. P. Qin, G. Q. Xie, X. D. Xu, J. Xu, P. Yuan, L. J. Qian. Dual-wavelength synchronous operation of a mode-locked 2-µm Tm:CaYAlO4 laser. Opt. Lett., 40, 356(2015).
[8] J. Wang, Y. Wang, B. Li, D. Feng, J. Lu, Q. Luo, P. Li. Dual-wavelength laser speckle imaging to simultaneously access blood flow, blood volume, and oxygenation using a color CCD camera. Opt. Lett., 38, 3690(2013).
[9] H. W. Chu, Z. B. Pan, X. Wang, S. Z. Zhao, G. Q. Li, H. Q. Cai, D. C. Li. Passively Q-switched Tm:CaLu0.1Gd0.9AlO4 laser at 2 µm with hematite nanosheets as the saturable absorber. Opt. Express, 28, 16893(2020).
[10] P. Loiko, J. M. Serres, X. Mateos, M. Aguiló, F. Díaz, L. Zhang, Z. Lin, H. Lin, G. Zhang, K. Yumashev, V. Petrov, U. Griebner, Y. Wang, S. Y. Choi, F. Rotermund, W. Chen. Monoclinic Tm3+:MgWO4: a promising crystal for continuous-wave and passively Q-switched lasers at ∼2 µm. Opt. Lett., 42, 1177(2017).
[11] Y. Zu, J. Guo, Q. Hao, F. Zhang, C. Wang, J. Liu, B. Wang. Graphdiyne as a saturable absorber for 2-µm all-solid-state Q-switched laser. Sci. China Mater., 64, 683(2020).
[12] W. Liu, Y. Zu, Y. Wang, Z. Zhang, J. Liu, L. Su. Active Q-switching operation of a Tm:SrF2 single crystal fiber laser near 2 µm. Opt. Mater. Express, 11, 2877(2021).
[13] Y. L. Yang, S. Z. Zhao, T. Li, W. C. Qiao, B. M. Ma, L. Guo, K. J. Yang, H. K. Nie, B. T. Zhang, R. H. Wang, J. L. He, Y. C. Wang. High-peak-power Q-switched 1988 nm bulk laser based on an electro-optical La3Ga5SiO14 modulator. Appl. Opt., 59, 2616(2020).
[14] L. Guo, S. Z. Zhao, T. Li, K. J. Yang, W. C. Qiao, D. C. Li, G. Q. Li, S. Y. Zhang, J. T. Bian, L. H. Zheng, L. B. Su, J. Xu. Diode-wing-pumped electro-optically Q-switched 2 µm laser with pulse energy scaling over ten millijoules. Opt. Express, 26, 17731(2018).
[15] F. Wang, H. Huang, H. Chen, Y. Bao, Z. Li, D. Shen. GSA and ESA dual-wavelength pumped 2.3 µm Tm:YLF laser on the 3H4→3H5 transition. Chin. Opt. Lett., 19, 091405(2021).
[16] S. S. Cai, J. Kong, B. Wu, Y. H. Shen, G. J. Zhao, Y. H. Zong, J. Xu. Room-temperature cw and pulsed operation of a diode-end-pumped Tm:YAP laser. Appl. Phys. B, 90, 133(2008).
[17] T. L. Feng, K. J. Yang, S. Z. Zhao, W. C. Qiao, J. Zhao, D. C. Li, G. Q. Li, T. Li, L. H. Zheng, J. Xu, Q. G. Wang, X. D. Xu, L. B. Su. An acoustic-optically Q-switched Tm:SSO laser at 2 µm. Opt. Commun., 336, 20(2015).
[18] Z. G. Wang, C. W. Song, Y. F. Li, Y. L. Ju, Y. Z. Wang. CW and pulsed operation of a diode-end-pumped Tm:GdVO4 laser at room temperature. Laser Phys. Lett., 6, 105(2009).
[19] V. Petit, J. L. Doualan, P. Camy, V. Menard, R. Moncorge. CW and tunable laser operation of Yb3+ doped CaF2. Appl. Phys. B, 78, 681(2004).
[20] Y. X. Wang, S. Z. Wang, J. Y. Wang, Z. H. Zhang, Z. Zhang, R. R. Liu, Y. Q. Zu, J. Liu, L. B. Su. High-efficiency ∼2 µm CW laser operation of LD-pumped Tm3+:CaF2 single-crystal fibers. Opt. Express, 28, 6684(2020).
[21] M. E. Doroshenko, K. A. Pierpoint, O. K. Alimov, A. G. Papashvili, V. A. Konyushkin, A. N. Nakladov. Formation of Tm-Y centers in CaF2-YF3:Tm3+ solid-solution crystals. J. Lumin., 208, 475(2019).
[22] S. Renard, P. Camy, A. Braud, J. L. Doualan, R. Moncorge. CaF2 doped with Tm3+: a cluster model. J. Alloys Compd., 451, 71(2008).
[23] X. Guo, J. Wang, D. Jiang, X. Qian, C. Zhang, Y. Zu, J. Liu, B. Mei, L. Su. Photoluminescence property and widely tunable, efficient 2 µm laser in Tm:Ca1−xGdxF2+x single crystals. Opt. Commun., 435, 5(2019).
[24] Z. Zhang, X. S. Guo, J. Y. Wang, C. Zhang, J. Liu, L. B. Su. High-efficiency 2 µm continuous-wave laser in laser diode-pumped Tm3+,La3+:CaF2 single crystal. Opt. Lett., 43, 4300(2018).
[25] Y. Q. Zu, X. S. Guo, J. J. Liu, J. Liu, L. B. Su. Passively Q-switched operation of a novel Tm3+, La3+ co-doped CaF2 single crystal near 2 µm. Infrared Phys. Technol., 102, 103010(2019).
[26] L. C. Kong, Z. Qiao, G. Q. Xie, Z. P. Qin, B. B. Zhao, H. Yu, L. B. Su, J. G. Ma, P. Yuan, L. J. Qian. Spectroscopic characteristics, continuous-wave and mode-locking laser performances of Tm,Y:CaF2 disordered crystal. Opt. Express, 25, 21267(2017).
[27] G. Cai, Y. Ju, B. Yao, W. Liu, X. Duan, T. Dai. Acousto-optically cavity dumped Tm:YAG laser with 54 ns pulses at 200 kHz repetition rate. Opt. Express, 22, 9942(2014).
[28] T. Feng, K. Yang, S. Zhao, J. Zhao, W. Qiao, T. Li, L. Zheng, J. Xu. Broadly wavelength tunable acousto-optically Q-switched Tm:Lu2SiO5 laser. Appl. Opt., 53, 6119(2014).
[29] X. Liu, K. Yang, S. Zhao, T. Li, G. Li, D. Li, X. Guo, B. Zhao, L. Zheng, L. Su, J. Xu, J. Bian. Kilo-hertz-level Q-switched laser characteristics of a Tm,Y:CaF2 crystal. Opt. Mater. Express, 7, 4352(2017).
[30] Y. Wen, H. Zhang, L. Zhang, S. Li, C. Wu, G. Jin. 1.99 micron Tm:YAP acousto-optical Q-switch laser. IOP Conf. Ser.: Mater. Sci. Eng., 563, 032007(2019).
[31] U. Sheintop, E. Perez, D. Sebbag, P. Komm, G. Marcus, S. Noach. Actively Q-switched tunable narrow bandwidth milli-Joule level Tm:YLF laser. Opt. Express, 26, 22135(2018).
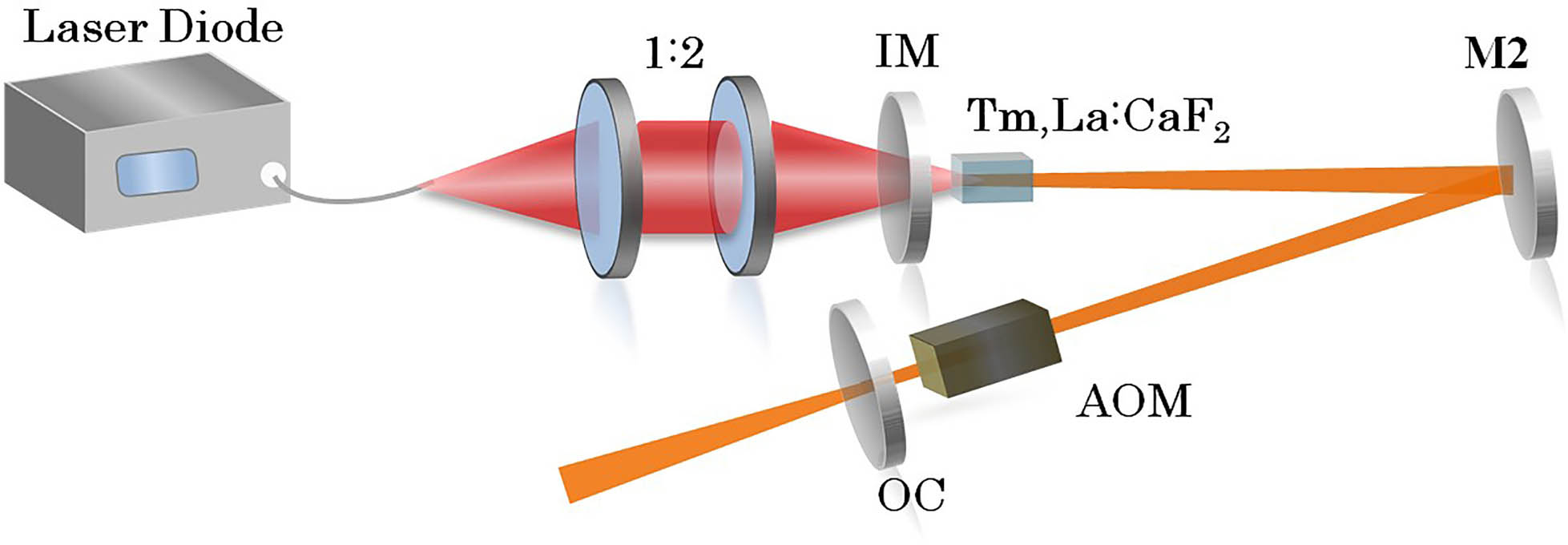
Set citation alerts for the article
Please enter your email address