Abstract
In this paper, we demonstrated SiNx/AlN/GaN metal-insulator-semiconductor high electron mobility transistors (MIS-HEMTs) with low noise and high linearity, by in-situ growth of SiNx gate dielectrics on ultra-thin barrier AlN/GaN heterostructure. Deep-level transient spectroscopy (DLTS) shows a traps-level depth of 0.236 eV, a capture cross-section of 3.06×10-19 cm-2, and an extracted interface state density of 1010-1012 cm-2eV-1, which confirms that the grown SiNx can reduce the interface state. The devices exhibit excellent DC, small signal, and power performance, with a maximum saturation output current (Idmax) of 2.2 A/mm at the gate voltage (Vgs) of 2 V and the gate length of 0.15 μm, a maximum current cutoff frequency (fT) of 65 GHz, a maximum power cutoff frequency (fMAX) of 123 GHz, a minimum noise figure (NFmin) of the device of 1.07 dB and the gain of 9.93 dB at 40 GHz. The two-tone measurements at the Vds of 6V, yield a third-order intermodulation output power (OIP3) of 32.6 dBm, and OIP3/Pdc of 11.2 dB. Benefited from the high-quality SiNx/AlN interface, the MIS-HEMTs exhibited excellent low noise and high linearity, revealing its potential in applications of millimeter waves.Introduction
Gallium nitride (GaN) based devices have been widely researched due to their advantages of high electron mobility,high breakdown voltage and high thermal conductivity,which can simplify the system of low-noise amplifiers and satisfy the requirements of circuit integration.
With the development of communication technology,the need of higher frequency for large bandwidth and lower noise is increasing[1].The size of the device is continuously scaling down as its working frequency rises,and the short channel effect that eventually appears weakens the capacity of the gate to control the channel. Traditional AlGaN/GaN HEMTs often reduce the aspect ratio by etching the gate slot to suppress the short channel effect. However,the etching process results in damages on the surface of AlGaN,which leads to the current collapse effect of the device and worsen the noise performance of the device. Therefore,the research of AlN thin barrier devices with stronger polarization effects in the field of noise has a certain value. Compared with AlGaN/GaN HEMTs,AlN/GaN HEMTs have stronger polarization and wider conduction band gap for lower probability of electrons entering the barrier layer in the channel. On this basis,gate dielectrics can effectively improve the interface quality of the device and further improve the noise performance. The selection of gate dielectrics and growth methods are crucial for device performance. In 2014,X. Lu [2] of the Hong Kong University of Science and Technology used MOCVD technology to grow SiNx gate dielectric layer in situ on AlN/GaN heterojunction,the measurement showed that the SiNx/AlN interface state presented 1011-1012 cm-2eV-1 low trap state densities. Therefore,MOCVD in situ growth of gate dielectric has certain significance for the research and development of low-noise devices.
At present,the research of GaN low-noise devices not only focuses on the improvement of the material growth and manufacturing processes,but also on the optimization of the device materials and structures,such as the choice of barrier layer materials,the optimization of heterostructure structure,etc. France F. Medjdoub et al. [1] adopted the AlN/GaN/AlGaN double-heterostructure structure on Si-based GaN,with a gate length of 80 nm,and achieved a gain of 7.5 dB and a minimum noise figure of less than 1 dB at 36 GHz in 2012. The article published in NANO LETTERS in 2020 by Choi Woojin et al. [3] reported the purpose of Fin field effect transistor (Fin-FET) applied to millimeter wave low noise amplifiers to obtain greater transconductance and reduce knee point voltage by shortening the source/drain spacing. The measurement showed that the device had a minimum noise figure of 2.2 dB at 30 GHz,and the linearity of the low-noise device was measured at 30 GHz with OIP3/Pdc ≥ 8.2 dB.
In this paper,based on the GaN process,AlN/GaN heterostructure was grown epitaxially on SiC substrate,and SiNx was grown in situ as gate dielectrics by MOCVD,high-quality gate dielectric material was obtained,and good interface characteristics were achieved. In addition,the AlN/GaN MIS-HEMTs with good noise performance applied in the millimeter wave frequency band were obtained by combining ohmic contact and the T-gate fabrication process. Deep-level transient spectroscopy (DLTS) technology was used to character the information of the device's gate dielectric material. Besides,the electrical properties such as DC output,small signal,noise,gain and linearity were evaluated.
1 Experiments
1.1 AlN/GaN heterostructure design
The short channel effect of the conventional AlGaN/GaN HEMT device is more apparent as the operating frequency of the device rises,and the gate length gradually shortens. In contrast,the thin barrier AlN material can achieve a higher density in two-dimensional electron gas with the AlN thickness of only 3-5 nanometers due to a stronger polarization effect.
The energy bands of SiNx/AlN/GaN heterostructure with different AlN thicknesses were simulated. The simulation result of the heterostructure is shown in Fig. 1(a),Fig. 1(b) demonstrates the relationship between the 2DEG density and the AlN barrier thickness. It is challenging to create the two-dimensional electron gas when the AlN thickness is 3 nm because no clear potential well forms. However,the potential well becomes increasingly apparent as the barrier thickness rises. As the thickness of the barrier layer increases,the 2DEG surface density rapidly increases and gradually approaches saturation. When the thickness of the AlN barrier is selected as 5 nm,the simulation results show that the 2DEG surface density is 1.98 × 1013 cm-2.
Thin barrier AlN/GaN heterostructure can be employed to satisfy millimeter wave application requirements without the necessity for etching,preventing the noise degradation and damage brought on by the etching process. Nevertheless,due to the lattice mismatch between the AlN and GaN materials up to 2.4%,the excessive thickness of AlN leads to the excessive stress of the material,and the strain relaxation is easy to crack during the growth of the material,which seriously affects the quality of the material. The barrier layer was chosen to be 5 nm,taking into account the actual application and the concentration of the two-dimensional electron gas,and the SiNx cap layer was generated in-situ to shield the AlN barrier layer from oxidation,contaminants,and water vapor adsorption.
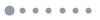
Figure 1.(a) Simulation diagram of SiNx/AlN/GaN heterostructure;(b) SiNx/AlN/GaN 2DEG density and heterostructure energy band simulation diagram
1.2 Main structure of device
The low-noise device is shown in Fig. 2(a),this paper used MOCVD in-situ growth technology to grow SiNx gate dielectrics,high quality dielectric material was obtained,and robust SiNx/AlN interface was achieved. The main function of the gate dielectric material is as follows: Firstly,due to the good insulation and high-quality SiNx/AlN interface characteristics of in-situ grown SiNx,it reduces the probability of electrons tunneling the barrier layer and injecting into the channel,reducing the collision of carriers in the channel,thus reducing the alloy scattering,and improving the mobility; Secondly,in-situ grown SiNx improves the interface quality of the AlN barrier layer to suppress the interface state,thus reducing gate leakage and noise. SiC with a high lattice matching degree and high thermal conductivity was selected as the substrate layer of the device. In order to further reduce the interface tension and thermal mismatch caused by lattice mismatch,the AlN nucleating layer is grown,which can reduce the current collapse caused by interface mismatch,defect or trap effect,and reduce the noise of the device. Finally,the passivation layer of SiNx can reduce the leakage current of the gate and increase the concentration of two-dimensional electron gas. In order to satisfy the millimeter-wave operation requirements of the device,the 150 nm T-shaped gate is adopted. As shown in Fig. 2(b) below,the gate resistance is reduced by increasing the gate cap structure to reduce the noise.
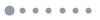
Figure 2.(a) Scanning electron microscopy(SEM) image of the two-finger device;(b) "T" gate structure of SEM
In this paper,after SiNx was grown in situ on AlN/GaN heterojunction using MOCVD technology,the source and drain metal Ti/Al/Ni/Au was evaporated on the epitaxial layer,and then annealed at 850 ℃ in N2 atmosphere for 50 seconds to form the source and drain ohmic contact; ion implantation isolation process was adopted to effectively isolate the active region; using three-layer photoresist technology and electron beam lithography technology to complete the manufacture of T-shaped gate; completing the metal wiring,using PECVD technology to deposit the SiNx passivation layer; making the air bridge and the thinning and back hole of the device.
2 Results and discussions
2.1 Surface state measurement
DLTS is an effective means to detect impurities and defects in the semiconductor at a deep level,which can obtain lots of information such as trap concentration and capture cross section [4].
In order to determine the defect information of gate dielectric interface states in MIS-HEMTs,constant capacitance deep level transient spectroscopy (CC-DLTS) technique was used to detect electron capture and emission processes near gate dielectric. Transient testing of devices in the temperature range of 10-400 K was completed using the Phystech GmbH FT-1030 DLTS system with a capacitance measurement frequency of 1 MHz,combined with the accompanying Lakeshore test stand.
In the CC-DLTS test mode,the SiNx/AlN/GaN MIS diode was biased at the pulse height UH = UP-UR = 3.5 V to extract the interface information of the device. The Arrhenius analysis and fitting curve are shown in Fig. 3(a),and the energy level depth of the defect EC-ET is about 0.23±0.01 eV. The capture cross section is about 3.05×10-19 cm2,and the density distribution of extracted interfacial state Nss is shown in Fig. 3(b) below. It can be seen from the figure that the extracted interfacial state concentration Nss decreases from 3×1011 cm-2eV-1 at T=10 K to 1.5×1010 cm-2eV-1 at T=400 K. With the increase of the pulse width tp,the signal has a small change range. Combined with the defect energy level information obtained by Arrhenius linear fitting,the measured trap concentration is the interface states at the SiNx/AlN interface in MIS structure.
In 2021,Fuqiang Guo[5] et al. in Microelectronics Institute of Chinese Academy of Sciences used in situ low-damage NH3/N2 remote plasma RPP technology to improve the surface morphology and improve the interface quality of the gate dielectric. They also used PEALD technology to deposition SiNx gate dielectrics and developed SiNx/AlGaN/GaN MIS-HEMTs. CC-DLTS measurements showed that the interfacial state concentration Nss decreased from 1×1013 cm-2eV-1 to 3.4×1011 cm-2eV-1 after RPP. In contrast,in this paper,the AlN thin barrier without etching process and MOCVD technology was used to grow SiNx gate dielectrics in situ,the interface damage and traps were effectively reduced while simplifying the process steps,and the interface concentration Nss was reduced by 1-2 orders of magnitude.
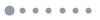
Figure 3.Measurements of device surface:(a) fitting curve of Arrhenius analysis;(b) density distribution of interfacial Nss
2.2 The DC and small-signal RF measurements
The semiconductor parameter analyzer was used to measure DC,small signal and other basic electrical properties of the device with 50 µm gate width and 4 µm source-drain spacing. The specific results are shown in Fig. 4.
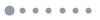
Figure 4.Basic electrical test of the device: (a) output I-V curves; (b) transfer and transconductance curves; (c) Vds = 6 V transconductance, first and second derivative curves of transconductance with respect to Vgs; (d) small signal test curve
From the current and voltage measurements in Fig. 4(a),it can be seen that the maximum saturation current of Vgs = 2 V is 2.2 A/mm,and the on-resistance (Ron) is 1.89 Ω·mm,due to the strong polarization effect of the thin barrier AlN,the device exhibites an excellent current output capacity. As can be seen in Fig. 4(b) of the transfer characteristics of the device,When Vds is 6 V,the maximum transconductance of the device is 503 mS/mm,and it can be seen from the comparison in the figure that the transconductance of Vds = 6 V has a larger transverse swing,indicating that the device has better linearity and stronger signal transfer ability under this bias condition. Under the condition of Vds being 6 V,the first-order and second-order derivatives of the trans-conductance gate-source voltage (Vgs) are obtained,and the curves of Gm,Gm′and Gm″are shown in Fig. 4(c),where the bias conditions corresponding to the intersection of the second derivative Gm " and the coordinate axes show good linearity and noise [7]. Subsequently,the measurement point Vgs = -4.1 V nearest to the intersection point was selected for the subsequent noise measurement.
Under the condition of Vds being 6 V and Vgs being -3 V,the frequency characteristics of the single-finger device were obtained by scanning the S parameter from the frequency range of 0.1-40 GHz. The measurements showed that the maximum current cutoff frequency (fT) of the device was 65 GHz and the maximum power cutoff frequency (fMAX) was 123 GHz under this measurement condition,which ensured the application requirements of the device in the millimeter wave frequency band.
2.3 Noise measurements
In order to better evaluate the noise performance of the device,PNA-X vector network analyzer was used based on the cold source method to perform on-chip measurements on the minimum noise figure and gain of the device with a gate width of 50×2 µm and source-drain spacing of 2.4 µm. The DC bias conditions were drain voltage (Vds) of 9 V,gate voltage (Vgs) of -4.1 V,and measurement frequency range was 8-40 GHz. The measurement results are shown in Fig. 5(a) below. The experimental results showed that NFmin of the device satisfied the linear fitting relationship with the measurement frequency. NFmin was 1.07 dB and the gain was 9.93 dB at 40 GHz,which showed superior noise and gain performance. On this basis,the gate voltage of -4.1 V was
maintained,and the noise and gain of the device at 30 GHz and 40 GHz were measured by changing Vds (1-10 V). The changes are shown in Fig. 5(b). The gain of the device tended to increase steadily with the rapid increase of Vds,while NFmin remained at a relatively low level. NFmin of Vds in the middle range (3-9 V) was stable at 0.85 dB at 30 GHz,and the low noise performance of 1.25 dB was also maintained at 40 GHz.
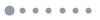
Figure 5.(a) Noise and gain curves of fixed bias devices; (b) variation curves of noise and gain with source-drain voltage
2.4 Linearity measurement
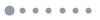
Figure 6.(a) Measurements of linearity of devices at Vds = 3 V; (b) measurements of devices at Vds = 6 V
Vds(V) | Zload | OIP3(dBm) | OIP3/Pdc(dB) | Gain(dB) |
---|
3 | 72.7+0.8*j | 28.5 | 7.3 | 8.2 |
6 | 62.5+57.6*j | 32.4 | 11.2 | 8.4 |
Table 1. Bias conditions and results for dual tone measurements
As a crucial component of RF reception front end,the anti-interference capability of the low noise device itself is the key index to evaluate the performance of the device. Therefore,the two-tone linearity of the device was measured in this paper. Based on the load traction system,a PNA-X vector network analyzer was used to provide signals with a frequency interval Δf of 1 MHz and a center frequency of 30 GHz. Devices with the gate width of 50×2 µm and source-drain spacing of 2.4 µm were also selected. In order to compare,the devices were biased at Vds = 3 V and 6 V,and static working current was 40 mA/mm.
It can be seen from the figure that the input power of the device is in the range of -10 dBm to10 dBm. When Vds = 3 V,OIP3 was 28.5 dBm and OIP3/Pdc was 7.3 dB. When the device was biased at Vds = 6,the maximum OIP3 of the device was 32.6 dBm,and OIP3/Pdc had better linearity than 11 dB at-10 dBm input power.
In order to better evaluate the performance of SiNx/AlN/GaN MIS-HEMTs,such as noise,gain and linearity,parameters selected in this paper are compared with those reported at home and abroad,as shown in Table 2 . At present,there are relatively few articles at home and abroad that pay attention to the noise and linearity of low noise devices at the same time. The SiNx/AlN/GaN MIS-HEMTs reported in this paper not only have the same linearity level as those at home and abroad,but also have excellent noise performance.
Paper | Frequency /(GHz) | NFmin/(dB) | Gain/(dB) | OIP3/(dBm) | OIP3/Pdc |
---|
2012[1] | 36 | 0.97 | 7.5 | - | - |
2019[6] | 30 | - | - | 35 | 11.4 |
2020[7] | 30 | - | 12.7 | 32 | 15 |
2020[3] | 30 | 2.2 | 5.0 | >31 | >8.2 |
2022[8] | 30 | 1.9 | 10 | 40 | 20 |
this paper | 30 | 0.85 | 10.75 | 32.60 | >11 |
40 | 1.07 | 9.97 | - | - |
Table 2. Comparison of performance of low noise devices reported for Millimeter-wave
3 Conclusions
In this paper,AlN/GaN MIS-HEMTs applied in the millimeter wave band were fabricated. Based on the basic structure and fabrication process of the device,the MIS-HEMTs with high quality interface states were obtained by using MOCVD in situ growth technology to prepare epitaxial SiNx gate dielectrics. At the same time,the trap information of SiNx/AlN interface of the device was obtained by CC-DLTS measurement. The traps-level depth was 0.236 eV and the capture cross-section was 3.06×10-19 cm-2,the extracted interface state density was 1010 to 1012 cm-2eV-1 .The device with a gate length of 0.15 µm had Idmax of 2.2 A/mm at Vgs of 2 V,the peak Gm was 506 mS/mm. ft and fMax of the device at Vds of 6 V were 65 and 123 GHz,respectively. The results of DC and small signal showed that the device had good output,transfer characteristics and application potential in the millimeter wave band. Benefited from the strong polarization effect of AlN/GaN and the high-quality interface state of SiNx/AlN,the devices have excellent noise performance and linearity. NFmin of the device was 1.07 dB and the gain was 9.93 dB at 40 GHz. When Vds was 6 V,the third-order intermodulation output power (OIP3) of the device was 32.6 dBm,and OIP3/Pdc reached 11.2 dB. Compared with the noise and linearity of low noise devices reported at present,some performance improvements have been made in this paper. In conclusion,the MIS-HEMTs fabricated in this paper based on SiNx/AlN/GaN heterostructure have excellent performances such as low noise and high linearity,and are high-performance devices working in the RF front end.
References
[1] F Medjdoub, Y Tagro, M Zegaoui et al. Sub-1-dB Minimum-Noise-Figure Performance of GaN-on-Si Transistors Up to 40 GHz. Electron Device Letters, IEEE, 33, 1258-1260(2012).
[2] X Lu, J Ma, H Jiang et al. Low trap states in in situ SiNx/AlN/GaN metal-insulator-semiconductor structures grown by metal-organic chemical vapor deposition. Applied Physics Letters, 105, 287-305(2014).
[3] Choi Woojin, Renjie Chen, Levy Cooper et al. Intrinsically Linear Transistor for Millimeter-Wave Low Noise Amplifiers. Nano letters, 20, 2812-2820(2020).
[4] Y Yao, Q Jiang, S Huang et al. Identification of bulk and interface state-induced threshold voltage instability in metal/SiNx(insulator)/AlGaN/GaN high-electron-mobility transistors using deep-level transient spectroscopy. Japanese Journal of Applied Physics, 119, 233502(2021).
[5] F Guo, S Huang, X Wang et al. Suppression of interface states between nitride-based gate dielectricss and ultrathin-barrier AlGaN/GaN heterostructure with in situ remote plasma pretreatments. Applied Physics Letters, 118, 093503(2021).
[6] M. Guidry, B. Romanczyk, H. Li et al. Demonstration of 30 GHz OIP3/PDC > 10 dB by mm-wave N-polar Deep Recess MISHEMTs. 2019 14th European Microwave Integrated Circuits Conference (EuMIC), 64-6(2019).
[7] P Shrestha, J F Buckwalter, U K Mishra et al. High Linearity and High Gain Performance of N-Polar GaN MIS-HEMT at 30 GHz. IEEE Electron Device Letters, 1-1(2020).
[8] M. Guidry, P. Shrestha, Wenjian Liu et al. Improved N-polar GaN mm-wave Linearity, Efficiency, and Noise, 291-294(2022).