Tae-Hyun Park, Sung-Moon Kim, Eun-Su Lee, Min-Cheol Oh, "Polymer waveguide tunable transceiver for photonic front-end in the 5G wireless network," Photonics Res. 9, 181 (2021)

Search by keywords or author
- Photonics Research
- Vol. 9, Issue 2, 181 (2021)
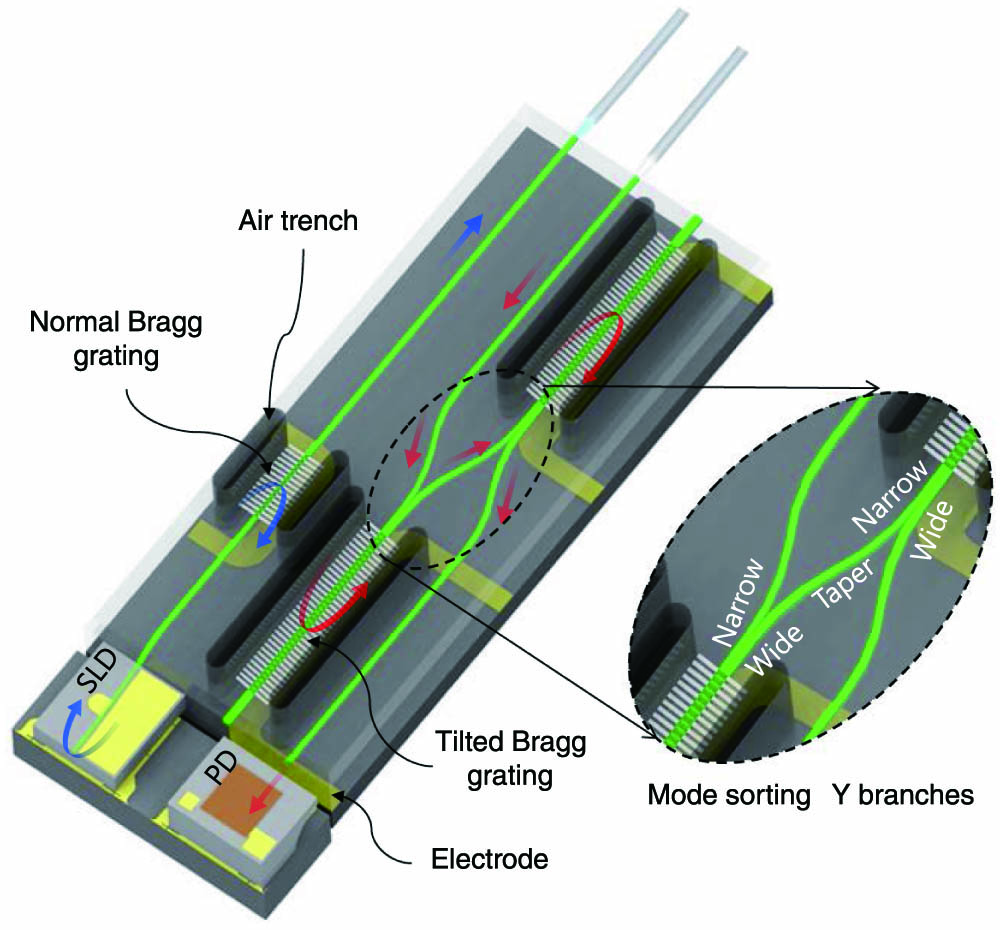
Fig. 1. Schematic diagram of the tunable transceiver consisting of a tunable laser and tunable wavelength filter based on polymer waveguide Bragg reflectors. The tunable laser consists of an SLD gain chip attached at the end of the polymer waveguide with a Bragg grating at the other end. The tunable wavelength filter has two stages: an inclined Bragg grating as well as the mode-sorting Y branches.
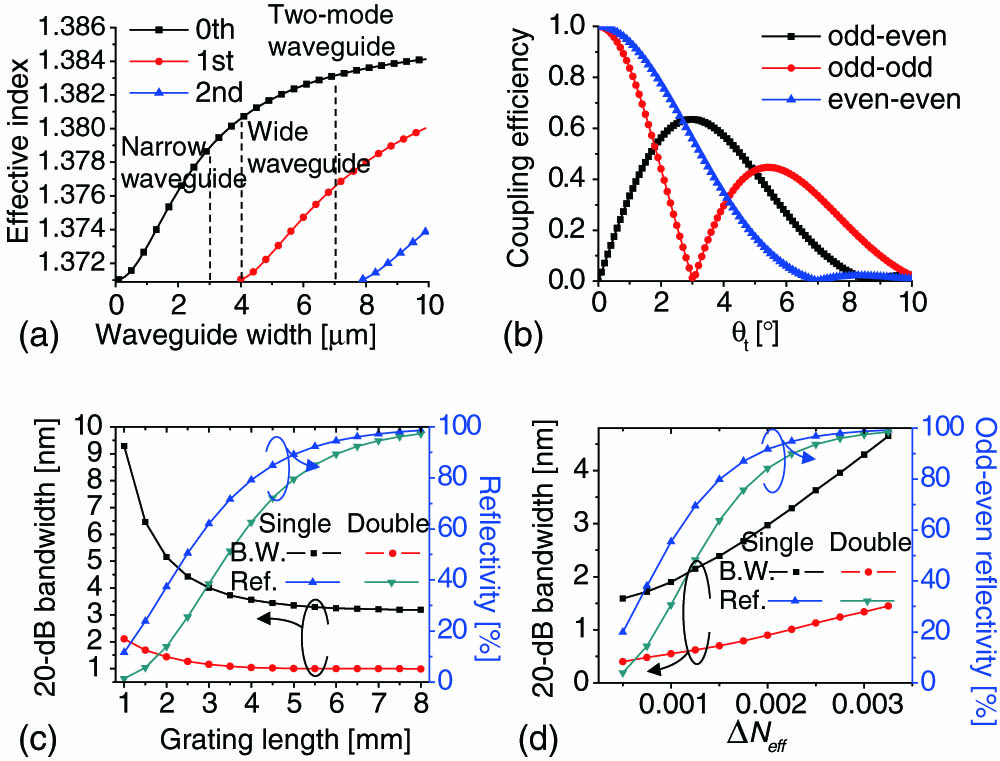
Fig. 2. Design results of the proposed device. (a) The effective index of the guided mode is calculated according to the waveguide width to find the single-mode and two-mode conditions. (b) Coupling efficiencies calculated as functions of the tilt angles between the odd–even modes η oe , odd–odd modes η oo , and even–even modes η ee . (c) Reflectivity and 20 dB bandwidth for odd–even coupling along the grating length, compared for single and double reflections. (d) 20 dB bandwidth and reflectance calculated as functions of the effective index difference Δ N eff due to the Bragg-grating thickness modulation.
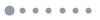
Fig. 3. Schematic of the fabrication procedure of the proposed two-stage connected wavelength-tunable filter and tunable wavelength laser. The two devices can be fabricated using the same fabrication procedure. The device cross section is shown along the red dashed single dotted line.
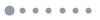
Fig. 4. (a) Microscopic photograph of optical waveguide pattern in the asymmetric Y-branch region, where 3, 4, and 7 mm waveguides are defined by etching through the Cr metal mask. (b) SEM photograph of fifth-order Bragg-grating pattern fabricated on top of the waveguide core through contact photolithography. (c) Microscopic photograph of air trenches and electrode pad opened by using the oxygen plasma etching.
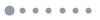
Fig. 5. (a) Tunable laser output spectrum measured with an OSA when 50 mA current was applied and (b) lasing spectra measured step by step while gradually increasing the power applied to the Bragg-grating electrode, in which the wavelength-tuning efficiency was 0.207 nm/mW and the power fluctuation was less than 1 dB.
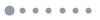
Fig. 6. (a) Reflection spectrum of the double-reflection tunable wavelength filters after the adjustment of two slightly different reflection spectra by applying a bias voltage when the two gratings have slightly different properties. (b) Reflection spectrum of another device with improved fabrication uniformity where the two Bragg gratings have identical reflection spectrum. (c) Polarization dependence of the reflection spectrum for the TE and TM modes, where only 0.08 nm of polarization dependence was observed. (d) Reflection spectra measured by increasing the heating power in steps. (e) Wavelength change drawn with respect to the input power, where linear wavelength tuning was achieved with a wavelength-tuning efficiency of 68.5 nm/W and 20 dB bandwidth was maintained below 0.8 nm.
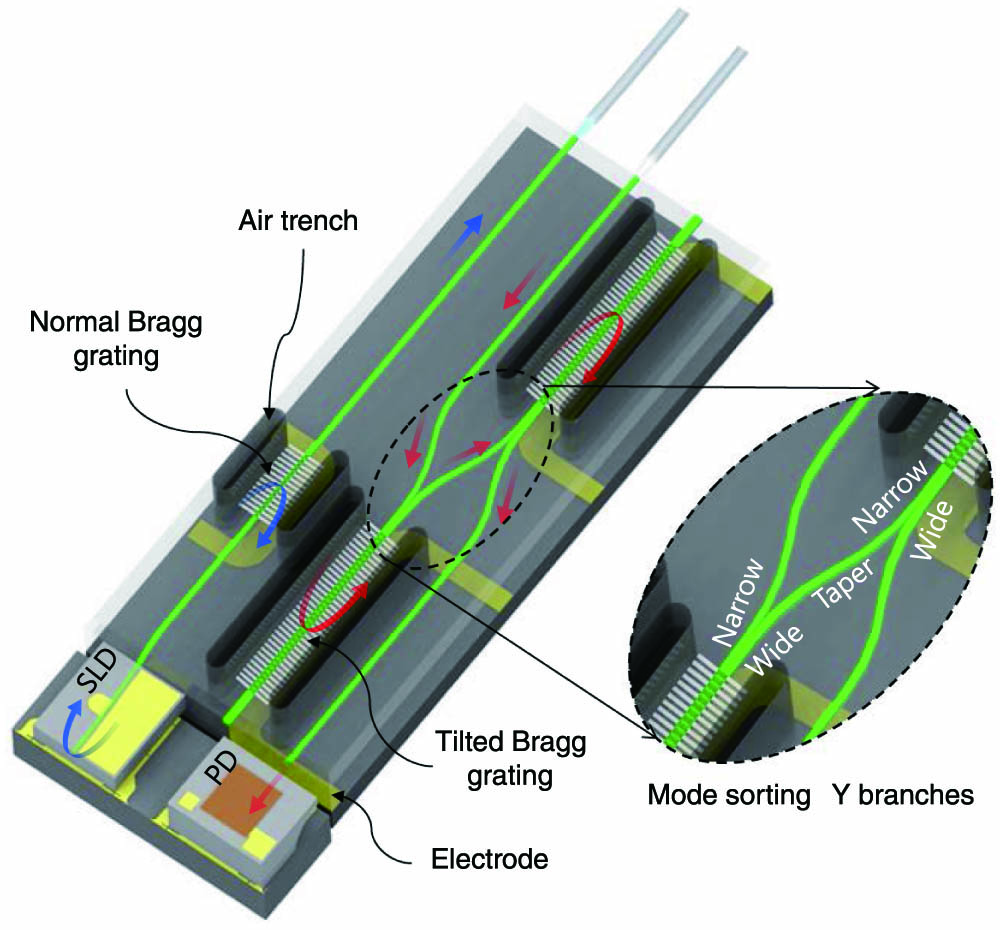
Set citation alerts for the article
Please enter your email address