
- Photonics Research
- Vol. 9, Issue 2, 181 (2021)
Abstract
1. INTRODUCTION
The recent explosive expansion of web-based video platform services has been unceasingly increasing data traffic and has become a driving force in the development of next-generation communication technologies. Wavelength-division-multiplexed (WDM) optical communication technology, which provides advantages such as high bandwidth, protocol transparency, and scalability, has become an important technology not only in metro-core networks but also in the fronthauls connecting radio-access networks [1,2]. Unlike core networks, fronthaul WDM networks require cost-effective network equipment, for which low-cost WDM transceivers are crucial [3]. Currently, the WDM system for the 5G fronthaul is set to meet the transmission distance of 10 km by using 10 Gbit/s transmission rate, 100 GHz channel spacing, and 48 wavelength channels in the C, L, and O bands. For this purpose, tunable laser technology has matured, while tunable filters are not yet available, making it difficult to find inexpensive tunable transceivers. By developing an integrated optic tunable filter with high performance, an integrated tunable transceiver could be realized to reduce the burden for deploying tunable wavelength transceivers for 5G fronthaul networks.
To provide the light sources of various wavelengths for WDM, a tunable laser with low inventory burden and low price is an attractive option [4–8]. The tunable laser in charge of each channel is set to operate at different wavelengths and is modulated using the information to be transmitted. The multiplexed wavelength signals arriving at the receiving end are demultiplexed using a thin-film filter or an arrayed waveguide grating (AWG). In early WDM optical communication systems with a small number of wavelength channels, thin-film filters were mainly used because of the simplicity and high production yield [9]. As the number of wavelength channels increased, AWG became more suitable for demultiplexing a large number of wavelength channels. However, the AWG separates each wavelength into a different fiber, so if the transmitter wavelength changes, the fiber must be reconfigured manually [10]. In contrast, with a tunable wavelength filter, the receiver can adjust the filtering wavelength simultaneously without manually switching the fiber.
Various approaches for implementing tunable filters include micro-electromechanical system-based Fabry–Perot (FP) filters, FP devices using the electroclinic effect of liquid crystals, acousto-optic filters using mode coupling, fiber Bragg gratings with piezoelectric actuators, and ring resonators with thermal tuning [11–16]. However, the above devices are not suitable for laser integration to produce compact tunable transceivers. In this work, we propose a polymeric optical waveguide tunable transceiver enabling the integration of a tunable laser and a tunable filter on a single chip. Both polymer lasers and filters include Bragg gratings with different structures, and they are fabricated simultaneously through a compatible fabrication process. Because the polymer has high thermo-optic (TO) effect and strong thermal confinement, wide tuning of Bragg wavelength is available, and because of the simple device structure, wavelength control is easy and high production yield is achievable [17].
Sign up for Photonics Research TOC. Get the latest issue of Photonics Research delivered right to you!Sign up now
In order to demonstrate a high-performance tunable filter device for WDM systems, we have been working on various approaches. Apodized Bragg gratings fabricated using shadow masks were effective in reducing the reflection bandwidth and improving the side-mode suppression ratio (SMSR) [18]. Asymmetric Y-branches and tilted Bragg gratings were adopted to improve the device integrality without using external circulators [19]. To improve the SMSR by suppressing spurious mode coupling, a two-stage tilted Bragg grating was employed [20]. In this work, the performance of the tunable filter element is improved further for the purpose of demonstrating an integrated tunable transceiver. Air trenches and lower electrodes are introduced to extend the wavelength-tuning range to 40 nm, and a polymer with very low birefringence is adopted to resolve the polarization dependence [21]. In addition, by reducing the insertion loss of the device to less than 3 dB and implementing SMSR exceeding 40 dB, performance levels suitable for 5G fronthaul optical communication networks are demonstrated.
2. DESIGN OF TUNABLE TRANSCEIVERS CONSISTING OF TUNABLE LASERS AND TUNABLE FILTERS
Figure 1.Schematic diagram of the tunable transceiver consisting of a tunable laser and tunable wavelength filter based on polymer waveguide Bragg reflectors. The tunable laser consists of an SLD gain chip attached at the end of the polymer waveguide with a Bragg grating at the other end. The tunable wavelength filter has two stages: an inclined Bragg grating as well as the mode-sorting Y branches.
Figure 2.Design results of the proposed device. (a) The effective index of the guided mode is calculated according to the waveguide width to find the single-mode and two-mode conditions. (b) Coupling efficiencies calculated as functions of the tilt angles between the odd–even modes
Bragg gratings for tunable laser are designed using a transmission matrix method. To fabricate the first-order grating with a period of approximately 0.5 μm, laser interferometry is required. However, for the integration of the laser and filter elements on a single chip, as targeted in this work, Bragg gratings of different structures must be created simultaneously using conventional photolithography. Therefore, a fifth-order Bragg grating is adopted for the integration purpose. Given a waveguide width of 3.5 μm, a core thickness variation between 2.5 and 3.2 μm in the Bragg grating produces a reflectivity of 23% for a grating length of 1 mm. The free spectral range of the external cavity laser becomes 0.11 nm for the waveguide length of 0.4 and 0.66 mm in the SLD and Bragg grating, respectively.
The mode coupling efficiency of the tilted Bragg grating used in the tunable filter is calculated using the mode overlap integral [19,23]. The odd- and even-mode profiles of the two-mode waveguide with a width of 7 μm are calculated, and then the coupling efficiencies between the odd–even modes
To consider the modal crosstalk in the asymmetric Y-branch waveguide, the adiabatic mode evolution process is simulated using the beam-propagation method. For a branch with an angle of 0.3° and narrow and wide waveguide widths of 3 and 4 μm, respectively, we find that the crosstalk can be less than
In order to expand the wavelength-tuning range, it is necessary to prevent the radiation of the guided mode caused by the thermal gradient. The heater located above the waveguide creates a temperature gradient in the core of the optical waveguide, creating a vertical gradient of refractive index profile. In particular, the odd mode existing in the two-mode waveguide is severely affected by the index gradient, resulting in large waveguide loss [20]. To overcome this problem and increase the tuning range, we minimize the thermal gradient by adopting a bottom electrode and introducing an air trench structure on the sides of the heater [24,25].
3. FABRICATION OF TUNABLE LASERS AND TUNABLE FILTERS BASED ON POLYMER BRAGG GRATINGS
Figure 3.Schematic of the fabrication procedure of the proposed two-stage connected wavelength-tunable filter and tunable wavelength laser. The two devices can be fabricated using the same fabrication procedure. The device cross section is shown along the red dashed single dotted line.
Figure 4.(a) Microscopic photograph of optical waveguide pattern in the asymmetric Y-branch region, where 3, 4, and 7 mm waveguides are defined by etching through the Cr metal mask. (b) SEM photograph of fifth-order Bragg-grating pattern fabricated on top of the waveguide core through contact photolithography. (c) Microscopic photograph of air trenches and electrode pad opened by using the oxygen plasma etching.
To fabricate the fifth-order Bragg grating, 50 Å thick Au layer is deposited through sputtering and a Bragg-grating pattern is formed through contact photolithography using diluted AZ5206. The Bragg-grating pattern is transferred to the core layer through oxygen plasma etching. Figure 4(b) shows the scanning electron microscope (SEM) photograph of the Bragg grating formed with a modulation depth of 0.7 μm and a period of 2.8 μm. The upper cladding is formed with the same polymer as the lower cladding, and the total thickness of the polymer layer becomes 31 μm. After depositing Cr of 100 nm thickness, an air trench and an electrode pad are opened by plasma etching using AZ5214 photoresist as shown in Fig. 4(c). End-facet polishing is carried out with sandpapers starting from coarse to fine grit size, and optical-grade polishing is completed using diamond suspensions. After finishing the end-facet polishing, an SLD is butt-coupled to the chip to form the ECL.
4. MEASUREMENT OF POLYMER WAVEGUIDE DEVICE CHARACTERISTICS
Figure 5.(a) Tunable laser output spectrum measured with an OSA when 50 mA current was applied and (b) lasing spectra measured step by step while gradually increasing the power applied to the Bragg-grating electrode, in which the wavelength-tuning efficiency was 0.207 nm/mW and the power fluctuation was less than 1 dB.
Figure 6.(a) Reflection spectrum of the double-reflection tunable wavelength filters after the adjustment of two slightly different reflection spectra by applying a bias voltage when the two gratings have slightly different properties. (b) Reflection spectrum of another device with improved fabrication uniformity where the two Bragg gratings have identical reflection spectrum. (c) Polarization dependence of the reflection spectrum for the TE and TM modes, where only 0.08 nm of polarization dependence was observed. (d) Reflection spectra measured by increasing the heating power in steps. (e) Wavelength change drawn with respect to the input power, where linear wavelength tuning was achieved with a wavelength-tuning efficiency of 68.5 nm/W and 20 dB bandwidth was maintained below 0.8 nm.
High-NA fiber with a core diameter of 1.8 μm and NA of 0.350 was used to reduce the coupling loss between the optical fiber and the polymer waveguide. The coupling loss between the high-NA fiber and the single-mode fiber could be as low as 0.1 dB by performing an adequate splicing process producing a tapered fiber core. Through the cutback method, the propagation loss of the polymer waveguide was measured to be 0.32 dB/cm for the wavelength of 1550 nm. Then the tunable filter with a length of 3.6 cm was expected to have a propagation loss of 1.15 dB, and the coupling loss to the high-NA fiber was measured to be 0.35 dB/facet. The loss due to the two Bragg gratings was expected to be 0.5 dB, and the excess loss by the asymmetric Y branch was 0.3 dB. By controlling the input polarization with a fiber-optic polarization controller, the polarization dependence of reflection peak was measured to be 0.08 nm and the polarization dependent insertion loss was 0.3 dB as shown in Fig. 6(c).
Wavelength-tuning characteristics were measured by increasing the heating power in steps. As shown in Fig. 6(d), 50 channels were produced at 100 GHz channel spacing. The insertion loss variation over the entire tuning range was maintained below 1.5 dB, and the SMSR was above 25 dB. The wavelength change with respect to the input power was measured as shown in Fig. 6(e). Linear wavelength tuning was achieved with a tuning efficiency of 68.5 nm/W, and the 20 dB bandwidth was maintained below 0.8 nm.
5. CONCLUSION
Based on the unique characteristics of the polymer waveguide tunable Bragg grating, we demonstrated tunable lasers and tunable filters that could be integrated into a single chip. The external cavity tunable laser consisting of polymer Bragg grating and SLD exhibited an output power of 4.2 dBm and allowed 55 wavelength channels at a spacing of 100 GHz. The tunable filter consisting of a tilted Bragg grating and an asymmetric Y branch was designed in a two-stage cascaded structure to achieve narrow reflection bandwidth, high SMSR, and low adjacent-channel crosstalk. In particular, the introduction of an air trench and a bottom-electrode structure solved the problem of radiation in the two-mode optical waveguide, which was an obstacle to widening the wavelength-tuning range. In addition, by introducing perfluorinated LFR polymer with low birefringence, polarization independence, an essential condition for a wavelength filter device, was secured. The tunable filter exhibited a 20 dB bandwidth of 0.69 nm, wavelength-tuning range of 40 nm, and SMSR of 42 dB, and it satisfied all the specifications required for 5G WDM fronthaul application. It is the first demonstration toward monolithic single-chip tunable transceiver modules based on polymeric tunable Bragg gratings, and these devices would be highly useful for data-center communication networks.
Acknowledgment
Acknowledgment. This research was supported by a National Research Foundation of Korea (NRF) grant funded by the Korean government (MSIP).
References
[3] B. Skubic, G. Bottari, P. Öhlén, F. Cavaliere. The role of DWDM for 5G transport. European Conference on Optical Communication, 1-3(2014).
[14] F. Ye, H.-S. Lee, R. Schleicher. Compact high-resolution tunable optical filter using optical diffraction element and a mirror. U.S. patent(2011).
[15] T. Nakazawa, S. Taniguchi, M. Seino. Ti:LiNbO3 acousto-optic tunable filter (AOTF). Fujitsu Sci. Tech. J., 35, 107-112(1999).
[25] Z. Zhang, N. Keil. Thermo-optic devices on polymer platform. Opt. Commun., 362, 101-114(2016).
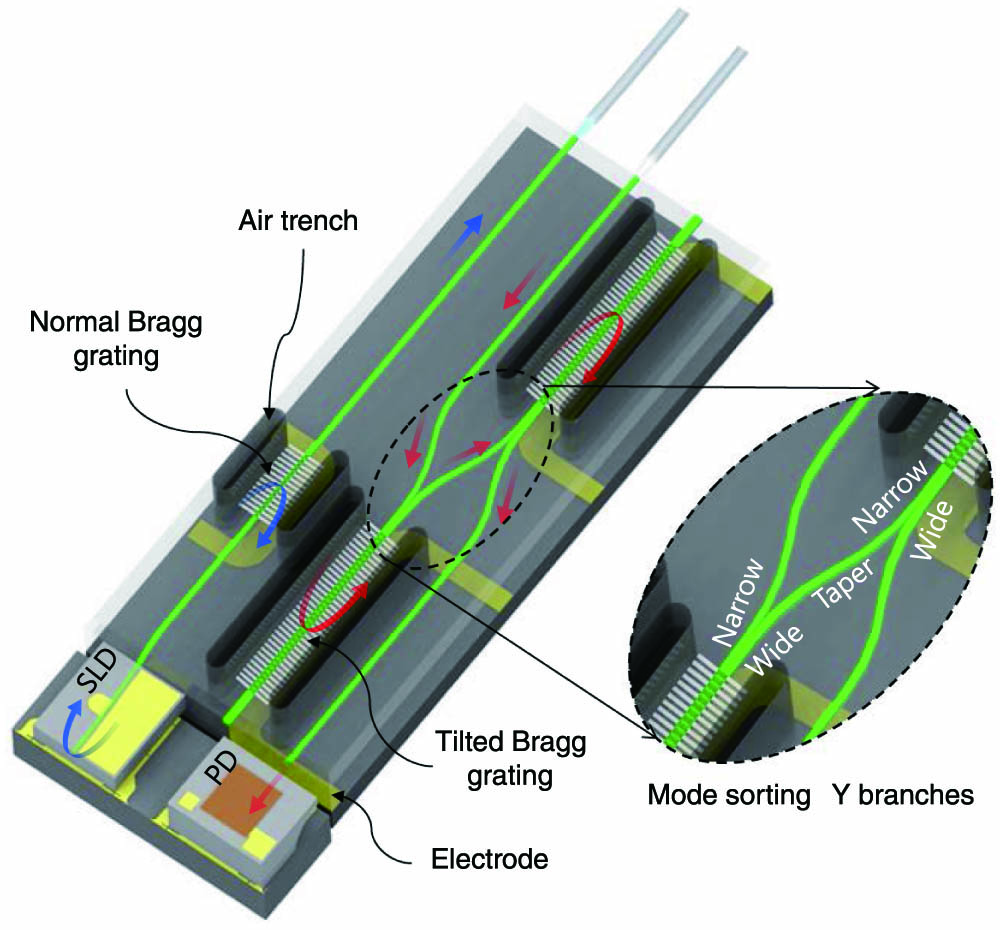
Set citation alerts for the article
Please enter your email address