Abstract
When a dielectric meta-atom is placed into a subwavelength metallic aperture, 20-fold enhanced electromagnetic transmission through the aperture is realized at the meta-atom’s resonant frequency. Additionally, when the incident electromagnetic power increases, thermal energy gathered by the meta-atom, which is converted from electromagnetic losses, can cause the meta-atom’s temperature to increase. Because of the high temperature coefficient of the meta-atom’s resonant frequency, this temperature increase causes a blueshift in the transmission peak. Therefore, this frequency-dependent enhanced electromagnetic transmission even produces a nonlinear effect at low incident powers. Over an incident power range from 0 to 20 dBm, measured and simulated spectra near the meta-atom’s resonant frequency show distinctly nonlinear transmission.1. INTRODUCTION
Because of huge breakthroughs in terms of transmission efficiency [1] and various potential applications in areas such as cavity quantum electrodynamics [2] and near-field optics [3], the enhanced transmission of electromagnetic waves through subwavelength metallic apertures has drawn extensive research attention [4–7] since the phenomenon was originally discovered. In addition to the surface plasmon resonance effect that exists on the surfaces of metallic films perforated with 2D aperture arrays, many research results have shown that the occurrence of other electromagnetic resonance modes can also cause transmission enhancement [8–14]. Meta-atoms [15–19], as the unit cells of metamaterials and metasurfaces, which exhibit extraordinary properties that are not available in natural media, are actually artificial subwavelength resonance structures that are based on metal or dielectric materials. Dielectric meta-atoms, due to their many advantages over metal meta-atoms, have been widely used in electromagnetically induced transparency [20], universal impedance matching of white light [21], perfect absorption for terahertz waves [22], and efficient optical wavefront control [23]. Because meta-atoms have both resonance and subwavelength properties simultaneously, their use should also provide outstanding performance levels and increase the amount of light that can pass through subwavelength apertures. In fact, our research group has made progress in this regard in recent years. For example, we have observed both dual band [24] and broadband [25] transmission enhancement produced by E-field coupling of split-ring resonators, magnetically [26] and thermally [27] tunable enhanced transmission using dielectric metamaterial resonators, and multimode [28] transmission using a thin dielectric rod.
However, despite the fact that the efficiency and the bandwidth of the waves that are transmitted through the subwavelength aperture can both be significantly improved through use of different types and combinations of meta-atoms, most of the research results to date have focused on linear transmission. To the best of our knowledge, there have been few reports in the literature on the nonlinear enhanced transmission characteristics of these subwavelength apertures when using meta-atoms. Because the electromagnetic fields that are localized in the meta-atoms are several orders of magnitude higher than the incident radiation fields [29–31], an obvious nonlinear effect should be generated on the subwavelength scale of the meta-atoms, despite the fact that the incident power is very low. Some nonlinear effects of metamaterials based on metal meta-atoms have been reported [32–35]; however, their transmission efficiency at the operating frequencies (resonant frequencies of the meta-atoms) is very low. In this work, we describe successful observation of a nonlinear enhanced transmission when a dielectric meta-atom constructed by a cubic calcium titanate particle is placed into a metallic subwavelength aperture. Under irradiation by electromagnetic waves at powers of no more than 20 dBm, the waves that are transmitted through the aperture experimentally demonstrate obvious nonlinear effects at operating frequencies near the resonant frequency. Electromagnetic simulations were also performed, and the results were generally consistent with the measured values. Therefore, the scheme in which meta-atoms are adopted to realize low-power nonlinear enhanced transmission is feasible.
2. PRINCIPLE
Figure 1 shows a schematic representation of low-power nonlinear enhanced transmission of electromagnetic waves through a subwavelength metallic aperture when the dielectric meta-atom is used. The aperture was constructed by forming a circular hole with a diameter of 5 mm at the center of a 1 mm thick copper plate, as shown in Fig. 1(a). For an incident electromagnetic wave at a frequency of approximately 12 GHz (), the operating wavelength is greater than the aperture diameter. Therefore, the proposed metal aperture is a subwavelength structure. In accordance with classical aperture theory, the electromagnetic wave that is transmitted through the aperture is considerably reduced. However, when a dielectric meta-atom cube with a side length of 2 mm is inserted into the aperture, as illustrated in Figs. 1(b) and 1(c), the transmission is enhanced at the resonant frequency of this meta-atom. To visualize the enhanced transmission produced by insertion of the dielectric meta-atom, the electric field intensity distributions at both the meta-atom’s resonant frequency and a nonresonant frequency were simulated with the CST Microwave Studio. In the simulations, the metal plate with the aperture is placed into a WR-90 rectangular waveguide, and the meta-atom is placed symmetrically at the center of the aperture. The electric field intensity distributions obtained at the nonresonant and resonant frequencies of the meta-atom are shown in Figs. 1(d) and 1(e), respectively. Figure 1(d) clearly shows that the electromagnetic waves that leak out into the other side of the aperture are very weak. This result is similar to the field distributions obtained for the aperture without the meta-atom (which are not shown here). Therefore, we use Fig. 1(d) to represent the electric field distributions of electromagnetic waves passing through a single aperture. The low transmission level is due to the discontinuous transmission structure that is formed by the presence of the obstacle, which is the metallic subwavelength aperture here. However, because the incident wave is strongly localized within the subwavelength range of the meta-atom at the resonant frequency of 11.73 GHz () and thus continues to pass unobstructively through the aperture, the transmitted wave intensity is enhanced extraordinarily and can even match the incident wave intensity if the dielectric loss is insignificant, as shown in Fig. 1(e). It should be noted that the level of miniaturization of the aperture is not just . In Ref. [36], we have realized a total broadband transmission through a subwavelength aperture with a diameter of 3 mm, which is about . Moreover, if we use the dielectric meta-atoms with a higher permittivity to realize transmission enhancement, the size of the metal aperture can be further reduced.
Sign up for Photonics Research TOC. Get the latest issue of Photonics Research delivered right to you!Sign up now
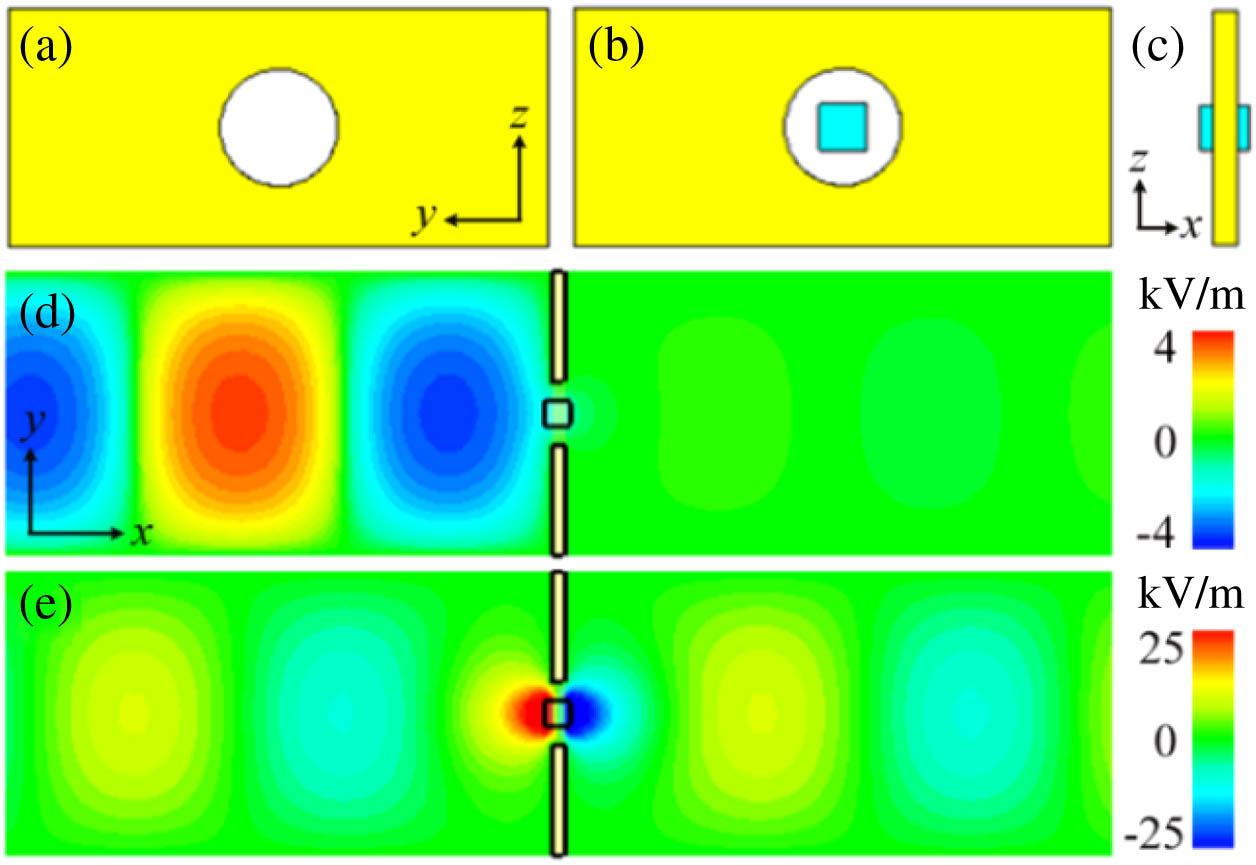
Figure 1.Schematic representation of a nonlinear enhanced electromagnetic transmission using a subwavelength metallic aperture with addition of a dielectric meta-atom. (a) Front view ( plane) of a subwavelength aperture at the center of a copper plate. (b) Front and (c) sectional views ( plane) of the subwavelength aperture with the inserted dielectric meta-atom. Electric field intensity distributions ( plane) at the (d) nonresonant frequency (11.5 GHz) and (e) resonant frequency (11.73 GHz) of the meta-atom when the metallic aperture with the added meta-atom is placed within the waveguide and the electromagnetic waves are excited from one of the waveguide ports.
In this study, the material combination used for the ceramic cube meta-atoms is calcium titanate doped with 1.5 wt.% of zirconium dioxide, and the permittivity, the loss tangent, and the temperature coefficient of permittivity of the fabricated ceramic meta-atoms are 118.55, 0.002, and , respectively. Because of the extremely strong electromagnetic fields that are localized in the subwavelength region and the specific degree of dielectric loss that occurs, part of the incident wave energy is converted into thermal energy and is stored in the meta-atom that has been placed in the aperture. Therefore, the operating temperature of the ceramic meta-atom will rise as the incident power increases, and because the ceramic meta-atom has a high negative temperature coefficient of permittivity, the permittivity and the resonant frequency of the meta-atom will fall and rise, respectively. This means that, with increasing incident electromagnetic power, the resonant frequency of the meta-atom moves distinctly toward higher frequencies. The transmission coefficient of the aperture when loaded with the ceramic meta-atom is highly sensitive around the resonant frequency of the meta-atom, and any tiny shift in the resonant frequency will also lead to a specific change in the transmission behavior. Therefore, use of a ceramic meta-atom with a high temperature coefficient of permittivity allows low-power nonlinear enhanced transmission of electromagnetic waves through subwavelength apertures to be realized.
Based on the analysis above, the principles of the nonlinear enhanced transmission phenomenon can be visualized as shown in Fig. 2. It shows that the transmission peak varies from the initial resonant frequency to a second resonant frequency and then to a third resonant frequency when the incident wave power increases gradually from to . If we compare the transmission values at incident powers , , and , we find that different nonlinear effects are obtained at the different frequencies. For example, at frequency , the difference is negative, while at frequency , the difference is positive. These results show that the transmission decreases and increases, respectively, with increasing incident power at the two different frequencies. However, at frequency , we find an increase and then a decrease of the transmission coefficient as the power increases. These results can thus direct us to obtain different nonlinear enhanced electromagnetic transmission values at different frequencies.
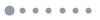
Figure 2.Principles of the nonlinear enhanced electromagnetic transmission properties of a subwavelength metallic aperture with an added dielectric meta-atom. Transmission coefficients , , and at incident powers of , , and , respectively.
3. RESULTS AND DISCUSSION
To verify the feasibility of the nonlinear transmission theories proposed above, the electromagnetic transmission spectra of the subwavelength metallic aperture with the added ceramic meta-atom, as depicted in Fig. 1, are studied quantitatively. The measured and simulated results are shown in Fig. 3. Simultaneously, to highlight the enhanced transmission properties produced by use of the meta-atom, the transmission spectrum of the single aperture alone was also studied. From Fig. 3(a), we see that the measured transmission coefficient is a very small value at approximately 0.035. We also know that the electromagnetic transmission of the single aperture does not vary with changes in the incident power (not shown here). However, when the ceramic meta-atom is inserted into the aperture, the transmission is generally enhanced and can reach as high as 0.7 at the resonant frequency of the meta-atom. Therefore, a near 20-fold enhancement of the electromagnetic transmission is realized. More importantly, the transmission peak clearly moves toward higher frequencies with increasing incident power. For example, at an incident power of 0 dBm, the transmission peak occurs at 11.73 GHz (). However, as the incident power is gradually increased to 20 dBm, the transmission peak shifts accordingly to 11.75 GHz (). We should emphasize here that each transmission spectrum was measured in the steady state, i.e., the ceramic particle had reached a thermal equilibrium state when each of the spectra was measured. Therefore, if the power is reduced from 20 dBm back to 0 dBm, the transmission curve will shift back in the exact same manner. Figure 3(a) also shows that the transmission peak decreases slightly with increasing incident power. This is caused by the increase in the dielectric losses that occurs as the meta-atom’s temperature rises.
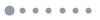
Figure 3.(a) Measured and (b) simulated transmission spectra of the single subwavelength metallic aperture and the same aperture with an inserted ceramic meta-atom at incident electromagnetic powers ranging from 0 to 20 dBm.
The simulated transmission spectrum of the single aperture alone is shown in Fig. 3(b) and is almost the same as the measured spectrum. In the electromagnetic simulation of the transmission spectra of the aperture with the added ceramic meta-atom, when the incident power is increasing, the permittivity and loss tangent values of the fabricated calcium titanate ceramic particle are the two main parameters that must be determined. These two parameters are known at room temperature; thus, the transmission spectrum of the aperture with the added meta-atom at an incident power of 0 dBm can be obtained easily. This is because the 0 dBm incident power is a very low power and does not cause any change in the material properties of the ceramic meta-atom. When the aperture is irradiated using other incident waves with powers of more than 0 dBm, these two material parameters can be determined by the following method.
The electromagnetic energy loss rate, i.e., the incident energy that is absorbed by the ceramic meta-atom per second, is given by [37] where is the operating frequency, is the maximum voltage of the vortex electric fields that are localized in the ceramic meta-atom (while assuming that the electric field strength in the meta-atom is uniform), is the permittivity of the fabricated ceramic, and is the dielectric loss tangent. We know that is proportional to the incident power , i.e., , where is the scale factor, and the absorbed electromagnetic power is actually determined with the transmitted and reflected powers. Therefore, Eq. (1) can be rewritten as where and are the transmission and reflection coefficients of the electromagnetic wave, respectively. In addition, the relationship between the resonant frequency of the meta-atom and the permittivity of the ceramic is given by [28] where is the light speed in vacuum, and is a constant that is related to the resonant mode of the meta-atom.
Because the permittivity and the loss tangent of the fabricated ceramic particle at room temperature and the measured transmission and reflection coefficients at each resonant frequency are all known, we can use Eqs. (2) and (3) above to calculate the permittivity and the loss tangent of the ceramic particle at any incident power of more than 0 dBm. Therefore, the transmission spectra at the different incident powers can be simulated with these calculated material parameters. The final electromagnetic simulation results are shown in Fig. 3(b), where the simulated spectra are basically the same as the measured spectra. The broader transmission bandwidth is caused by the loss tangent of the dielectric meta-atom, which is larger than the real value because Eq. (2) is derived on the condition that the metal waveguide and subwavelength aperture are lossless.
Next, we specifically demonstrate the nonlinear transmission characteristics of electromagnetic waves transmitted through the subwavelength aperture with the added ceramic meta-atom. From the basis of the measured and simulated results presented in Fig. 3, we can obtain the variation of the transmission coefficients with changes in the incident power at a specific frequency near the resonant frequency of the meta-atom, as shown in Fig. 4. The results indicate that different types of nonlinear transmissions occur at different frequencies. In Fig. 4(a), we see that a reduced nonlinear transmission is realized at 11.71 GHz (). For example, when the incident power is 0 dBm, the transmission coefficient is approximately 0.6. However, the coefficient is reduced distinctly to 0.4 when the incident power is increased to 20 dBm. In Fig. 4(b), we see that the transmission coefficient at 11.74 GHz () initially increases with increasing incident power, but the coefficient then starts to decrease when it reaches a maximum value of 10 dBm. In contrast with Fig. 4(a), the results depicted in Fig. 4(c) show that the transmission coefficient at 11.76 GHz () increases with increasing incident power. For example, at the incident power of 0 dBm, the transmission is approximately 0.5. When the incident power is increased to 20 dBm, the transmission is also increased to 0.6. Therefore, increased nonlinear transmission has been realized. In addition, to verify that the nonlinear transmission effects are introduced by the meta-atom and that they can only occur near the resonant frequency of the meta-atom, the transmission coefficient at 12 GHz (), i.e., away from the resonant frequency, was also measured, with results as shown in Fig. 4(d). We see that the transmission coefficient is approximately 0.13, which is a very low value, and the coefficient remains nearly unchanged with increasing incident power. Therefore, the ceramic meta-atom-based nonlinear enhanced transmission of electromagnetic waves through a subwavelength metallic aperture is verified.
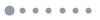
Figure 4.Transmission spectra of the subwavelength aperture with the added ceramic meta-atom over the incident power range from 0 to 20 dBm. Enhanced nonlinear transmission is shown at (a) 11.71 GHz, (b) 11.74 GHz, and (c) 11.76 GHz, while linear transmission is shown at (d) 12 GHz. Black lines represent the measured results; red lines represent the simulated results.
4. CONCLUSIONS
In summary, by placing a fabricated ceramic meta-atom with a high temperature coefficient of resonant frequency into a subwavelength metallic aperture, we have demonstrated nonlinear electromagnetic transmission at low incident powers of no more than 20 dBm. The measured and simulated results show that gradually reducing and increasing nonlinear transmission and convex-type nonlinear transmission can be realized at different frequencies close to the resonant frequency of the meta-atom. This proposed low-power nonlinear transmission scheme can broaden the application range of subwavelength apertures and can be used in many fields, including thermal sensors and filters.
References
[1] T. W. Ebbesen, H. J. Lezec, H. F. Ghaemi, T. Thio, P. A. Wolff. Extraordinary optical transmission through sub-wavelength hole arrays. Nature, 391, 667-669(1998).
[2] N. F. Yu, J. Fan, Q. J. Wang, C. Pflugl, L. Diehl, T. Edamura, M. Yamanishi, H. Kan, F. Capasso. Small-divergence semiconductor lasers by plasmonic collimation. Nat. Photonics, 2, 564-570(2008).
[3] J. A. Matteo, L. Hesselink. Fractal extensions of near-field aperture shapes for enhanced transmission and resolution. Opt. Express, 13, 636-647(2005).
[4] F. J. Garcia-Vidal, H. J. Lezec, T. W. Ebbesen, L. Martin-Moreno. Multiple paths to enhance optical transmission through a single subwavelength slit. Phys. Rev. Lett., 90, 213901(2003).
[5] W. L. Barnes, W. A. Murray, J. Dintinger, E. Devaux, T. W. Ebbesen. Surface plasmon polaritons and their role in the enhanced transmission of light through periodic arrays of subwavelength holes in a metal film. Phys. Rev. Lett., 92, 107401(2004).
[6] R. Gordon, A. G. Brolo, A. McKinnon, A. Rajora, B. Leathem, K. L. Kavanagh. Strong polarization in the optical transmission through elliptical nanohole arrays. Phys. Rev. Lett., 92, 037401(2004).
[7] K. J. K. Koerkamp, S. Enoch, F. B. Segerink, N. F. van Hulst, L. Kuipers. Strong influence of hole shape on extraordinary transmission through periodic arrays of subwavelength holes. Phys. Rev. Lett., 92, 183901(2004).
[8] F. J. Garcia-Vidal, L. Martin-Moreno, T. W. Ebbesen, L. Kuipers. Light passing through subwavelength apertures. Rev. Mod. Phys., 82, 729-787(2010).
[9] K. Aydin, A. O. Cakmak, L. Sahin, Z. Li, F. Bilotti, L. Vegni, E. Ozbay. Split-ring-resonator-coupled enhanced transmission through a single subwavelength aperture. Phys. Rev. Lett., 102, 013904(2009).
[10] A. B. Khanikaev, S. H. Mousavi, G. Shvets, Y. S. Kivshar. One-way extraordinary optical transmission and nonreciprocal spoof plasmons. Phys. Rev. Lett., 105, 126804(2010).
[11] K. B. Alici, E. Ozbay. Metamaterial inspired enhanced far-field transmission through a subwavelength nano-hole. Phys. Status Solidi, 4, 286-288(2010).
[12] Z. C. Ruan, M. Qiu. Enhanced transmission through periodic arrays of subwavelength holes: the role of localized waveguide resonances. Phys. Rev. Lett., 96, 233901(2006).
[13] R. Marques, J. Martel, F. Mesa, F. Medina. Left-handed-media simulation and transmission of EM waves in subwavelength split-ring-resonator-loaded metallic waveguides. Phys. Rev. Lett., 89, 183901(2002).
[14] L. Zhou, C. P. Huang, S. Wu, X. G. Yin, Y. M. Wang, Q. J. Wang, Y. Y. Zhu. Enhanced optical transmission through metal-dielectric multilayer gratings. Appl. Phys. Lett., 97, 011905(2010).
[15] N. Meinzer, W. L. Barnes, I. R. Hooper. Plasmonic meta-atoms and metasurfaces. Nat. Photonics, 8, 889-898(2014).
[16] R. Tsu, M. A. Fiddy. Generalization of the effects of high Q for metamaterials. Photon. Res., 1, 77-87(2013).
[17] K. E. Chong, L. Wang, I. Staude, A. R. James, J. Dominguez, S. Liu, G. S. Subramania, M. Decker, D. N. Neshev, I. Brener, Y. S. Kivshar. Efficient polarization-insensitive complex wavefront control using Huygens’ metasurfaces based on dielectric resonant meta-atoms. ACS Photon., 3, 514-519(2016).
[18] D. R. Smith, J. B. Pendry, M. C. Wiltshire. Metamaterials and negative refractive index. Science, 305, 788-792(2004).
[19] V. M. Shalaev. Optical negative-index metamaterials. Nat. Photonics, 1, 41-48(2007).
[20] Y. M. Yang, I. I. Kravchenko, D. P. Briggs, J. Valentine. All-dielectric metasurface analogue of electromagnetically induced transparency. Nat. Commun., 5, 5753(2014).
[21] K. Im, J. H. Kang, Q. H. Park. Universal impedance matching and the perfect transmission of white light. Nat. Photonics, 12, 143-149(2018).
[22] X. Y. Liu, K. B. Fan, I. V. Shadrivov, W. J. Padilla. Experimental realization of a terahertz all-dielectric metasurface absorber. Opt. Express, 25, 191-201(2017).
[23] K. E. Chong, I. Staude, A. James, J. Dominguez, S. Liu, S. Campione, G. S. Subramania, T. S. Luk, M. Decker, D. N. Neshev, I. Brener, Y. S. Kivshar. Polarization-independent silicon metadevices for efficient optical wavefront control. Nano Lett., 15, 5369-5374(2015).
[24] Y. S. Guo, J. Zhou. Dual-band-enhanced transmission through a subwavelength aperture by coupled metamaterial resonators. Sci. Rep., 5, 8144(2015).
[25] Y. S. Guo, J. Zhou. Total broadband transmission of microwaves through a subwavelength aperture by localized E-field coupling of split-ring resonators. Opt. Express, 22, 27136-27143(2014).
[26] K. Bi, W. J. Liu, Y. S. Guo, G. Y. Dong, M. Lei. Magnetically tunable broadband transmission through a single small aperture. Sci. Rep., 5, 12489(2015).
[27] Y. S. Guo, H. Liang, X. J. Hou, X. L. Lv, L. F. Li, J. S. Li, K. Bi, M. Lei, J. Zhou. Thermally tunable enhanced transmission of microwaves through a subwavelength aperture by a dielectric metamaterial resonator. Appl. Phys. Lett., 108, 051906(2016).
[28] Y. S. Guo, J. Zhou, C. W. Lan, K. Bi. Resonance transmission of electromagnetic wave through a thin dielectric rod. Appl. Phys. Lett., 104, 123902(2014).
[29] J. C. Prangsma, D. van Oosten, R. J. Moerland, L. Kuipers. Increase of group delay and nonlinear effects with hole shape in subwavelength hole arrays. New J. Phys., 12, 013005(2010).
[30] N. Papasimakis, V. A. Fedotov, V. Savinov, T. A. Raybould, N. I. Zheludev. Electromagnetic toroidal excitations in matter and free space. Nat. Mater., 15, 263-271(2016).
[31] H. Cang, A. Labno, C. G. Lu, X. B. Yin, M. Liu, C. Gladden, Y. M. Liu, X. Zhang. Probing the electromagnetic field of a 15-nanometre hotspot by single molecule imaging. Nature, 469, 385-388(2011).
[32] M. W. Klein, C. Enkrich, M. Wegener, S. Linden. Second-harmonic generation from magnetic metamaterials. Science, 313, 502-504(2006).
[33] M. W. Klein, M. Wegener, N. Feth, S. Linden. Experiments on second- and third-harmonic generation from magnetic metamaterials. Opt. Express, 15, 5238-5247(2007).
[34] Y. Zeng, W. Hoyer, J. J. Liu, S. W. Koch, J. V. Moloney. Classical theory for second-harmonic generation from metallic nanoparticles. Phys. Rev. B, 79, 235109(2009).
[35] C. Ciraci, E. Poutrina, M. Scalora, D. R. Smith. Origin of second-harmonic generation enhancement in optical split-ring resonators. Phys. Rev. B, 85, 201403(2012).
[36] Y. S. Guo, J. Zhou, C. W. Lan, H. Y. Wu, K. Bi. Mie-resonance-coupled total broadband transmission through a single subwavelength aperture. Appl. Phys. Lett., 104, 204103(2014).
[37] W. Wang, Y. R. Qu, K. K. Du, S. A. Bai, J. Y. Tian, M. Y. Pan, H. Ye, M. Qiu, Q. Li. Broadband optical absorption based on single-sized metal-dielectric-metal plasmonic nanostructures with high-ϵ″ metals. Appl. Phys. Lett., 110, 101101(2017).