Abstract
We prepare coatings on different substrates by either electron-beam evaporation or dual ion-beam sputtering. The relative transmittances of the coatings are measured during the heating process. The coating microstructures are studied. Results indicate that the intensity and peak position of moisture absorption are closely related to the microstructures of the coatings. The formation of microstructures depends not only on the preparation process of the coatings but also on the substrate characteristics.Infrared lasers operating within the 2–3 μm wavelength range have various applications, such as in medical equipment[1,2], space remote sensing[3–5], optical communication[6], and gas detection. Growing demands in the fields of offensive and defensive space applications as well as strong field laser physics have led to the development of broadband, tunable, ultra-short, and ultra-intense mid-infrared lasers. A large coefficient of water absorption is a noteworthy property of this band. Relevant to this research field is the fact that different forms and hydrates of water considerably influence the optical properties, internal stress[7], and laser irradiation resistance of coatings[8]. However, published research conducted on their adsorption characteristics is uncommon. Adsorption characteristics can help us to deeply understand the mechanism pertaining to how moisture adsorption affects film performance. In this Letter, we report film deposition by two kinds of process on different substrates. The adsorption characteristics and microstructures of the films were detected and analyzed.
An infrared quartz material was selected and deposited on different substrates by electron-beam evaporation (EBE) and dual ion-beam sputtering (IBS). The substrates included fused silica, silicon crystal, and alumina crystal. The parameters of the coating processes are listed in Table 1.
Deposition Method | Coating Material | Substrate | Baking Temperature (°C) | Base Pressure (Pa) | O2 Pressure (Pa) | Thickness (μm) |
---|
EBE | SiO2 | Fused quartz, silicon crystal, and alumina crystal | 300 | 5×10−3 | 1×10−2 | 2 |
IBS | 70 | 1×10−4 | 4×10−2 | 2 |
Table 1. Parameters of the Coating Process
Spectra as a function of temperature were obtained with a Fourier-transform infrared (FTIR) spectrometer (Nicolet 6700, Thermo Fisher). The FTIR spectrometer had a diffuse reflectance and a high-temperature reaction chamber to observe the desorption process in real time. Scanning was repeated 32 times and the resolution was . The rate of heating was 10°C/min and the precision of the temperature control was 1°C.
Sign up for Chinese Optics Letters TOC. Get the latest issue of Chinese Optics Letters delivered right to you!Sign up now
The surface morphologies and coating roughness were determined by atomic force microscopy (AFM; Dimension 3100, Bruker Nano), which had a measurement error within 0.01 nm. Each test site on the samples was scanned over an area of . The cross sectional morphologies were observed by focused ion-beam (FIB) field-emission scanning electron microscopy (FESEM; Auiga, Zeiss). The resolution was 1.7 nm when the acceleration voltage was 1 kV.
The respective vibrations we observed from free liquid water, solid water, and adsorbed water are listed in Table 2 in order to compare the vibrations of these three types of water.
Spectral evolution of the coatings during desorption was indicative of different intensities and peak positions of the moisture absorption, as shown in Figs. 1 and 2.
Sample | Wavenumber (cm−1) | Wavelength (μm) | Vibration Mode | References |
---|
Water | 3280 | 3.05 | Stretching OH Symmetric, ν1 | [9] |
| 3490 | 2.86 | Stretching OH Asymmetric, ν3 | |
Solid phase of water | 3200 | 3.13 | OH stretching of ice structure | [10] |
Liquid phase of water | 3400 | 2.94 | OH stretching of liquid H2O structure | |
Mesoporous silica | 3400 | 2.94 | OH in free H2O | [11] |
| 3672 | 2.72 | OH in Si-OH | |
Mesoporous silicon oxide | 3230 | 3.10 | OH stretching of ice-like structure | [12] |
| 3400 | 2.94 | OH stretching of liquid H2O structure | |
Table 2. Water Vibrations in the Mid-Infrared Spectral Region
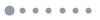
Figure 1.Variations in the FTIR spectra of coatings deposited by IBS as a function of heating temperature.
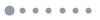
Figure 2.Variations in the FTIR spectra of coatings deposited by EBE as a function of heating temperature.
The relative transmittance of the coatings deposited by IBS slightly increased in intensity as the temperature increased. The increasing bands were between 3280 and (3.05 and 2.86 μm, respectively). This indicates a vibration mode of OH stretching pertinent to free (Table 2).
The relative transmittance of the coatings deposited by EBE strongly increased in intensity as the temperature increased. The increasing bands were between 3240 and (3.09 and 3.05 μm, respectively). This position corresponds to OH stretching of an ice-like structure (in accordance with the peak position in Table 2). The decreasing bands, at (2.71 μm), are attributable to heating-induced Si–OH production.
In order to determine why the relative transmittance changed, the surfaces and cross sectional morphologies of the samples were measured.
As shown in Fig. 3, the root-mean square (RMS) roughness of the substrates was small (no more than 0.61 nm). The surfaces of the fused quartz and silicon crystal were uneven, which imparted a larger RMS than that of the alumina crystal.
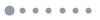
Figure 3.Surface structures of the different substrates.
Figure 4 shows surface structures of coatings deposited by IBS, and Fig. 5 shows the cross sectional morphology. The RMS roughness was small (no more than 0.49 nm). The coatings on the fused quartz and silicon crystal were uneven because the substrates were uneven. The coating was obviously dense (Fig. 5). Due to the dense structure, moisture could not enter the interior of the coatings and remained on the surface. The change of the relative transmittance was related to a close association between the water and the surface. Consequently, the relative transmittance of the coatings only slightly increased in intensity when we increased the temperature.
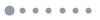
Figure 4.Surface structures of coatings deposited by IBS on different substrates.
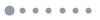
Figure 5.Scanning electron microscopy (SEM) cross sectional morphology of a coating fabricated by IBS.
Figure 6 shows surface structures of coatings deposited by EBE and Fig. 7 shows the cross-sectional morphology. The RMS roughness was more than 1 nm (Fig. 6). The microstructure was columnar and porous (Fig. 7) The RMS roughness was far more than that of the corresponding substrate due to the EBE-induced columnar structure[13]. Due to the columnar and porous structure, absorbed moisture entered the coatings and filled the pores along the columnar structure. Consequently, the relative transmittance of the coatings strongly increased in intensity in accordance with increasing temperature.
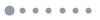
Figure 6.Surface structures of coatings deposited by EBE on different substrates.
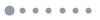
Figure 7.SEM cross sectional morphology of a coating fabricated by EBE.
The pore sizes of the coatings were analyzed through the “grain size” functional module in our AFM software. The threshold height was set as 2.50 nm. The mean pore sizes of the coatings were between 25 and 30 nm (Fig. 8). When the pore diameters were small (3–20 nm), the structure of in the mesoporous silicon oxide was affected by both near- and far-surface structures[11]. Therefore, the effects of the interfaces overlapped and were strengthened, causing the water molecules to become more ice-like. The overlap of the interface effect induced formation of a solid-water-solid system. When the pore diameters were large (30–50 nm), the structure of the water was only affected by the near-side surface. Hence, larger pores affected the molecular structure less than smaller pores.
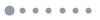
Figure 8.Mean pore sizes of the coatings prepared by EBE on different substrates.
As indicated in Fig. 8, the pore size of the coatings on the alumina crystal was less than that on the fused quartz and silicon crystal. Preliminary analysis suggested that this was attributable to the different coefficients of thermal expansion between the substrates (Table 3). During deposition, in which the baking temperature was increased up to 300°C, the coefficient of thermal expansion of the alumina crystal was larger than that of the other substrates. Thus, the contraction of the alumina crystal was more drastic during the cooling process, which led to a smaller pore size. Furthermore, the sharp contraction of the alumina crystal also led to a large amount of stress; thus, cracking and peeling of the coating on the alumina crystal could occur[14–16].
Material | Coefficient of Thermal Expansion (10−6 K) | References |
---|
Fused quartz | 0.5 | [17] |
Silicon crystal | 3.6 | [18] |
Alumina crystal | 7.3–8.1 | [18] |
SiO2 coating prepared by EBE | 0.4 | [19] |
Table 3. Coefficients of Thermal Expansion of the Substrates and Coating
In conclusion, we measure the FTIR spectra of coatings to investigate the forms and hydrates of in coatings through the heating process. Results show that moisture absorption is largely on the surface of dense coatings fabricated by IBS. Adsorption capacity is obviously low; the absorption peak is similar to that of free . For coatings that have a columnar and porous structure as induced by EBE, moisture enters the interior of the coatings and fills the pores along the columnar structure. Some Si–OH form due to the interaction between the water molecules and . Most of the water molecules become more ice-like due to the coating pore sizes. The mean sizes of the pores in the columnar structure are somewhat variable because of the thermal expansion coefficient of the substrates.
References
[1] M. Natali Cizmeciyan, H. Cankaya. Opt. Lett., 34, 3056(2009).
[2] Q. Wang. Opt. Lett., 34, 3616(2009).
[3] P. A. Budni, L. A. Pomeranz, M. L. Lemons, C. A. Miller, J. R. Mosto, E. P. Chicklis. J. Opt. Soc. Am. B., 17, 723(2000).
[4] P. A. Budni, M. L. Lemons, J. R. Mosto, E. P. Chicklis. IEEE J. Quantum Electron., 6, 629(2000).
[5] G. Renz, W. Bohn. Proc. SPIE, 6552, 655202(2007).
[6] J. Ren, R. Zhou, S. Lou. Chin. Opt. Lett., 12, 090605(2014).
[7] E. H. Hirsch. J. Phys. D, 13, 2081(1980).
[8] L. Na, W. Yingjian, Z. Ming. Chin. Phys. Lett., 27, 150(2010).
[9] T. Richard, L. Mercury, F. Poulet. J. Colloid Interface Sci., 304, 125(2006).
[10] G. E. Ewing. J. Phys. Chem. B, 108, 15953(2004).
[11] J. Abe, N. Hirano, N. Tsuchiya. J. Mater. Sci., 47, 7971(2012).
[12] D. B. Asay, S. H. Kim. J. Phys. Chem. B, 109, 16760(2005).
[13] J.-C. Zhang, L.-M. Xiong, F. Ming, H.-B. He. Chin. Phys. B, 22, 253(2013).
[14] T. Tan, J. Huang, M. Zhan, G. Tian, J. Shao, Z. Fan. Proc. SPIE, 5774, 555(2004).
[15] T.-Y. Tan, D.-W. Zhang, M.-Q. Zhan, J.-D. Shao, Z.-X. Fan. Chin. Phys. Lett., 22, 227(2005).
[16] Z. Deng, H. Gao, L. Xiao, H. He, Z. Fan, J. Shao. Chin. Opt. Lett., 5, 60(2007).
[17] H. Tada, A. E. Kumpel, R. E. Lathrop. J. Appl. Phys., 87, 4189(2000).
[18] W. M. Vim, R. J. Paff. J. Appl. Phys., 45, 1456(1974).
[19] C.-C. Lee, C.-L. Tien, W.-S. Sheu. Rev. Sci. Instrum., 72, 2128(2001).