Alexei V. Balakin, Jean-Louis Coutaz, Vladimir A. Makarov, Igor A. Kotelnikov, Yan Peng, Peter M. Solyankin, Yiming Zhu, Alexander P. Shkurinov, "Terahertz wave generation from liquid nitrogen," Photonics Res. 7, 678 (2019)

Search by keywords or author
- Photonics Research
- Vol. 7, Issue 6, 678 (2019)
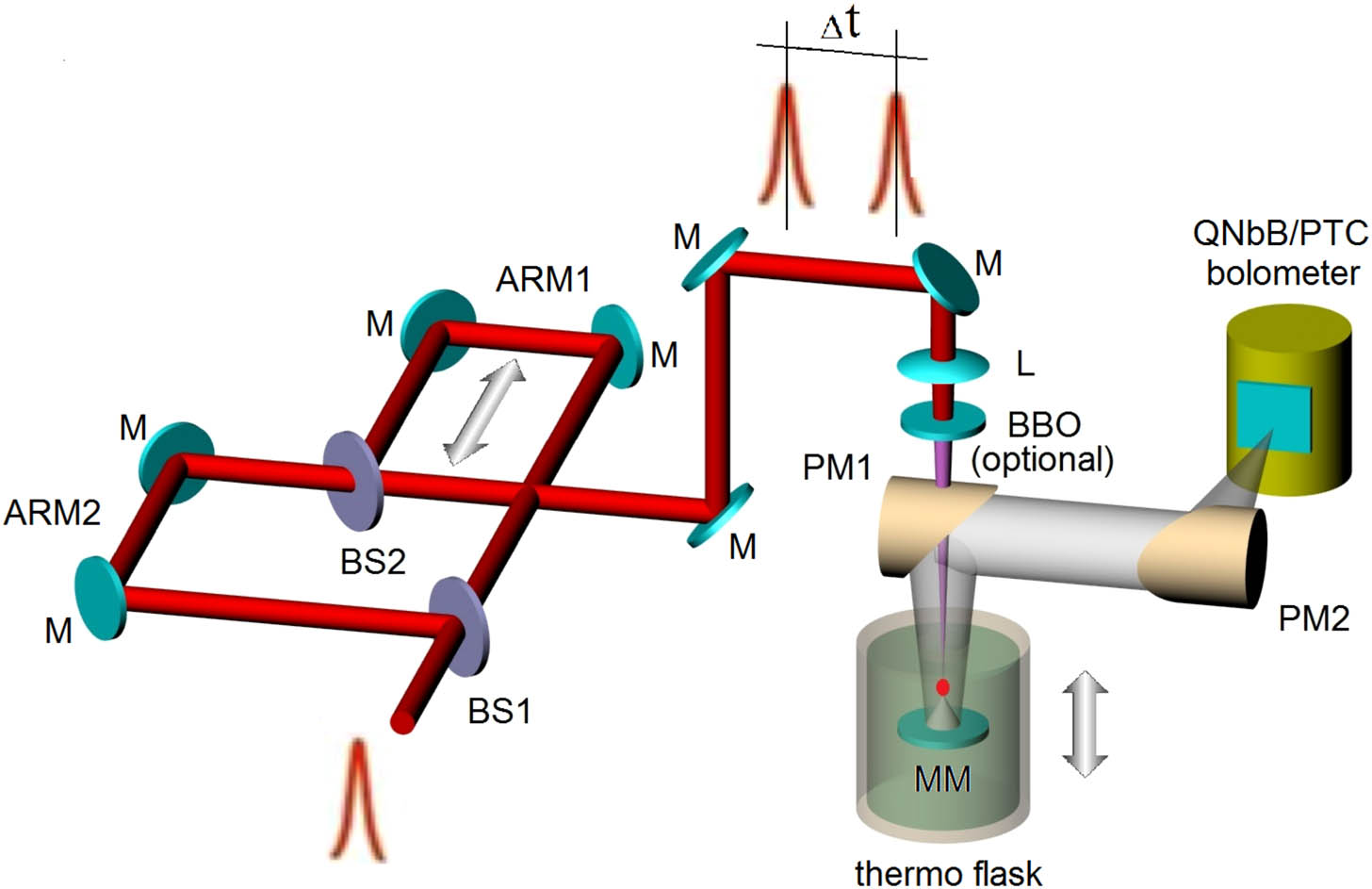
Fig. 1. Experimental setup. M–dielectric mirror; MM–metallic mirror; BS–beam splitter; λ / 2 –half-wave phase plate; L–lens; PM–off-axis parabolic mirror; BBO–β -barium borate crystal.
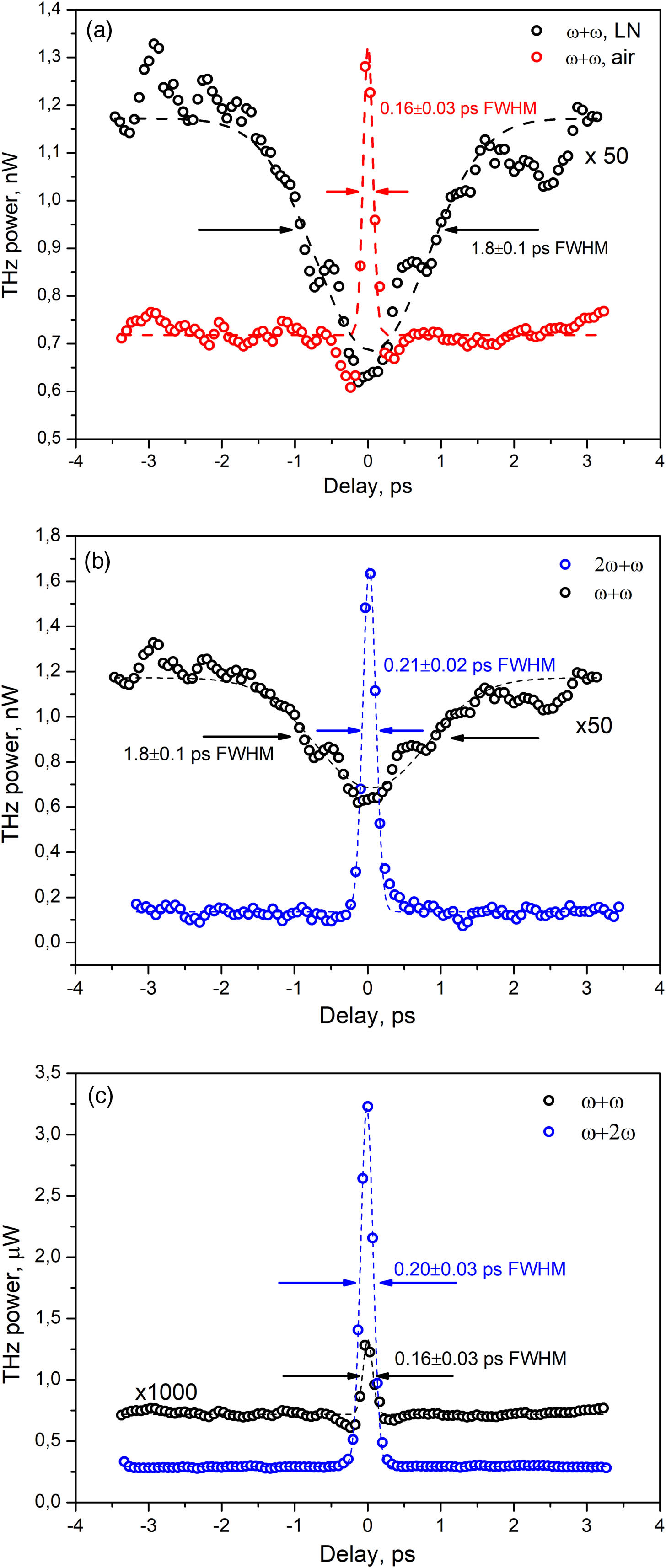
Fig. 2. Terahertz yield via the time delay between the laser pulses. (a) Single-color interaction in air (red circles) and in LN (black circles). (b) LN: single-color (black) and dual-color (blue) schemes. (c) Air: single-color (black) and dual-color (blue) schemes. Each curve is fitted by a simple Gaussian peak to get FWHM value.
![Dependence of terahertz pulse power on the beam waist position h in relation to the surface level of LN. Zero position of the abscissa axis corresponds to the surface of LN. Peak amplitude of the terahertz pulse power is measured in two beams’ dual-color regime [blue dots in Fig. 2(b)].](/Images/icon/loading.gif)
Fig. 3. Dependence of terahertz pulse power on the beam waist position h in relation to the surface level of LN. Zero position of the abscissa axis corresponds to the surface of LN. Peak amplitude of the terahertz pulse power is measured in two beams’ dual-color regime [blue dots in Fig. 2(b) ].
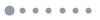
Fig. 4. Radiated terahertz energy versus laser pulse energy: solid squares with error bars show experimental data, and solid line stands for theoretical model. (a) Focal spot located in the air; (b) focal spot located in the liquid nitrogen.
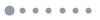
Fig. 5. Diagram of terahertz generation from “liquid” plasma under irradiation by a double-pulse beam.
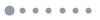
Fig. 6. Schematic representation of the ambipolar field effect on the terahertz yield. (a) Field formation by the first pulse and probing by the second one; (b) total terahertz yield from two pulses with variable delay. τ corresponds to the optical pulse duration, while τ 1 and τ 2 correspond to the characteristic times of ambipolar field formation and decay.
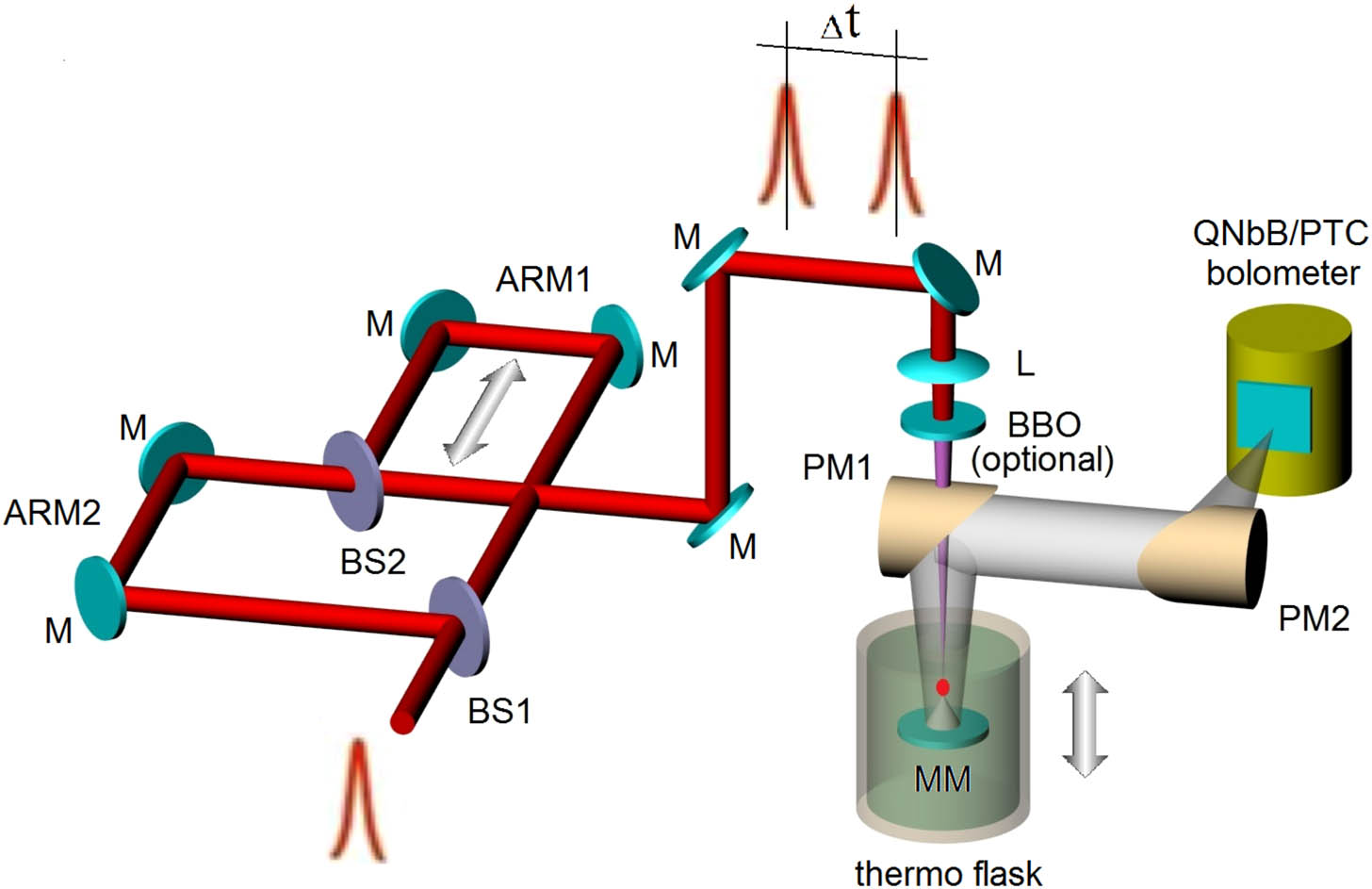
Set citation alerts for the article
Please enter your email address