
- Acta Photonica Sinica
- Vol. 50, Issue 8, 0850213 (2021)
Abstract
Keywords
0 Introduction
As a new generation of photovoltaic technology, organic solar cells have attracted extensive attention in recent years due to their advantages of low cost, light weight and solution processing[
In the field of material engineering, many methods were employed to improve the device performance, such as rational design for both donor and acceptor materials and the good matching between them[
In 2011, naphthalenedithiophene-based donor material was firstly introduced in the application of the photovoltaic device by Marks group[
In organic solar cells, photophysical processes leading to photocurrent generation are usually described as: 1) the active layer materials of organic solar cells absorb sunlight photons and consequently generate electron-hole (E-H) pairs (exciton); 2) the exciton diffuses toward the donor-acceptor (D-A) interface, where an intermediate Charge Transfer (CT) state is formed after an ultrafast CT reaction; 3) the CT state then further splits into free charges (Charged Separation, CS) by breaking the E-H pair binding energy under the driving force offered by the energy difference of the interface; 4) the free charges drift-diffuse in the bulk materials and are eventually collected by the cathode and anode electrodes. Each step is accompanied by loss processes, for example, incomplete photon absorption, exciton recombination, geminate recombination, bimolecular recombination and so on. As PCE is a measure of the ability to convert solar energy to electric energy through all the above photophysical processes, any factors that can influence the above photophysical processes could affect the final PCE.
It is generally believed that the higher charge separation yield, the more conducive to the generation of free charges, a more balanced carrier mobility and a longer lifetime of CS state are facilitated to the charge transport and collection, which positively contribute to the overall PCE. In short, PCE is a comprehensive measurement index, before further investigation on the photo-generated carrier dynamics, one cannot conclude an improved PCE is benefited from which photophysical processes. To study the effect of the side chain modification and SVA treatment on PCE of NDT-based ASM-OSCs from the aspect of dynamic, we applied femtosecond Transient Absorption (fs-TA) measurement on heterojunctions composed of NDT (NDT-Hex) as donors and PC71BM as acceptors. We extracted the lifetime of CS state and the CS yield through Global Analysis (GA) of TA spectra and discussed their contribution to the PCE upon side chain modification and SVA treatment.
1 Materials and methods
1.1 Sample preparation
The synthetic details and the spectroscopic characterization of materials used here have been reported elsewhere[
1.2 UV-Vis absorption spectra measurements
All steady-state spectra of samples were measured using Agilent Technologies Cary 60 UV-Vis spectrophotometer. The spectral range of the source is 200~1 100 nm.
1.3 Femtosecond pump-probe absorption spectroscopy
Fs-TA spectra were acquired on a home-build TA system. The output of a Ti:Saphire laser system (Astrella, Coherent company, The United States) was split to generate pump and probe beams. About 0.6 W of 800 nm laser pulse was introduced to a noncollinear optical parametric amplifier (Topas White, Light Conversion, Lithuania) to generate visible pump beam. About 3 mW of 800 nm laser pulse was focused onto a sapphire crystal to generate a supercontinuum and used as a probe beam. To eliminate polarization selection effects, the angle between the polarizations of pump and probe beams was set to the magic angle (54.7°). The transmitted probe beam through the sample was sent to a spectrometer and collected by a CCD. The delay time between pump and probe is controlled by a high-resolution delay stage. No significant sample degradation was observed throughout the TA experiments. Repeat measurements were done on several different spots for each sample to ensure the reliable results. Global analysis of the dispersion-corrected data was done using CarpetView software package.
1.4 Dynamics model and global analysis
The fs-TA data are analyzed globally using a target model based on the method discussed in detail in previous articles[
2 Experimental results and discussion
The chemical structures of NDT and NDT-Hex are shown in Fig. 1. Both molecules are comprised of an electron-rich NDT core and two electron-deficient units. The mainly structure difference of them is the hexyl group is introduced at
Figure 1.Chemical structure of NDT and NDT-Hex small molecule donors
Figure 2.Normalized UV-Vis absorption spectra
To unravel the effect of side chain modification and SVA treatment on the photoelectric processes in the heterojunction film, fs-TA spectroscopy was conducted on neat films and blend films. A low pump fluence was implemented to eliminate the effects of multiphoton absorption and annihilation processes between excitons and/or charges. All samples were measured under the same condition.
Figs. 3(a) and (d) show the TA carpet of neat films upon excitation with 610 nm optical pump. The pump here only excites the donor material, which is also confirmed by zero TA signal when the sample is replaced by neat PC71BM film. NDT and NDT-Hex shows similar TA response. Both of them are composed of one negative band and a part of positive band in the probe spectral region. The shape of negative band resembles the shape of the ground-state absorption, and thus this band is assigned to the Ground-State Bleaching (GSB) of donor material[
Figure 3.TA measurement results of neat films
Fig.4 shows the TA results of three heterojunction films (NDT:PC71BM, NDT-Hex:PC71BM and NDT-Hex:PC71BM-SVA). The spectral features of blend films are almost identical to those of neat films with one GSB band and part of ESA band. Fig. 4(g) and (h) presents the normalized TA traces of the three blend films at the probe wavelength of 640 nm and 720 nm. The TA signals of the blend film persist to the time scale longer than that in neat film, suggesting other competing processes, such as intermolecular charge transfer and charge separation in blend films. The TA traces in blend films shows that the decay of 640 nm is slowest for NDT-Hex:PC71BM film while the other two films show similar fast decay. In contrast, in the TA trace of 720 nm (ESA), NDT:PC71BM exhibits fastest dynamics decay and NDT-Hex:PC71BM and NDT-Hex:PC71BM-SVA show similar slow decay. Here, the initial EX state and the followed CT and CS states are severely overlapped in spectra, obviously, it’s difficult to conclude interconversion dynamics only from the overall TA traces.

Figure 4.TA measurement results of blend films
To address the above issue, Global Analysis (GA) procedure was applied to all the blend films based on the model described in the method part as shown in Fig.5(a) where three states were involved (EX state, CT state, CS state). The CT state refers to the situation in which the hole delocalized on the Highest Occupied Molecular Orbital (HOMO) of a donor molecule and the electron on the Lowest Unoccupied Molecular Orbital (LUMO) of an adjacent acceptor PC71BM molecule, while the electron-hole pairs are still strongly bound by coulomb interactions. After the CT state dissociated, the carriers can undergo several ultrafast hops, allowing them to separate before the CS state thermal relaxation and the carrier entering the normal polaron hopping regime. The formation of CT/CS states is usually fast, as a result, the other longer process (such as traps and triplet states) are not involved in the limited time window. Species-Associated Difference Spectra (SADS) and the kinetics of the corresponded components for NDT:PC71BM film are shown in Figs.5(b) and (c) as an example. The CT/CS spectral features basically match with EX but with a slight difference at ~650 nm. In CT/CS states, the distance between electrons and holes is already large, which minimize the binding energy of electron-hole pairs and leads to GSB peak red shifts[
The time constants from the GA fit for all blend samples are listed in Table 1. With these parameters, the CS yield was calculated by the equation of
NDT:PC71BM | NDT-Hex:PC71BM | NDT-Hex:PC71BM-SVA | |
---|---|---|---|
1.9±0.4 | 1.7±0.3 | 1.7±0.3 | |
22±7 | 20±6 | 20±6 | |
68±33 | 70±37 | 70±37 | |
1.4±0.2 | 2±0.5 | 0.40±0.03 | |
5.9±1.0 | 5.8±2.0 | 3.5±0.6 | |
157±60 | 280±180 | 134±48 | |
CS yield | 0.59±0.06 | 0.49±0.07 | 0.81±0.03 |
Table 1. Target fitting parameters and the corresponding CS efficiency obtained from the broadband transient absorption data of NDT-based blend films
3 Conclusion
Three heterojunction films (NDT:PC71BM, NDT-Hex:PC71BM and NDT-Hex:PC71BM-SVA) were investigated by TA spectroscopy. The dynamics results showed that the chemical modification and SVA treatment can alter several photophysical processes in the blend films. Solely focusing on one process cannot well explain the overall PCE. The highest CS yield and best balanced electron-hole mobility of NDT-Hex:PC71BM-SVA give rise to the largest PCE among the three blend films. The longer CS lifetime and better balanced electron-hole mobility of NDT-Hex:PC71BM leads to a higher PCE than that of NDT:PC71BM. This work shows that the CS yield, CS lifetime and electron-hole mobility are all important factor for PCE improvement, thus for the device optimization, these three factors should all be considered to ensure the PCE is improved essentially.
References
[1] G LI, R ZHU, Y YANG. Polymer solar cells. Nature Photonics, 6, 153-161(2012).
[2] Y SUN, G C WELCH, W L LEONG et al. Solution-processed small-molecule solar cells with 6.7% efficiency. Nature Materials, 11, 44-48(2012).
[3] J ZHAO, Y LI, G YANG et al. Efficient organic solar cells processed from hydrocarbon solvents. Nature Energy, 1, 15027(2016).
[4] C YAN, S BARLOW, Z WANG et al. Non-fullerene acceptors for organic solar cells. Nature Reviews Materials, 3, 18003(2018).
[5] Y CUI, H YAO, J ZHANG et al. Single-junction organic photovoltaic cells with approaching 18% efficiency. Advanced Materials, 32, 1908205(2020).
[6] Q LIU, Y JIANG, K JIN et al. 18% Efficiency organic solar cells. Science Bulletin, 65, 272-275(2020).
[7] J QIN, L ZHANG, C ZUO et al. A chlorinated copolymer donor demonstrates a 18.13% power conversion efficiency. Journal of Semiconductors, 42(2021).
[8] K JIN, Z XIAO, L DING. 69% PCE from organic solar cells. Journal of Semiconductors, 42(18).
[9] M B SALIM, R NEKOVEI, R JEYAKUMAR. Organic tandem solar cells with 18.6% efficiency. Solar Energy, 198, 160-166(2020).
[10] A MISHRA, P BAEUERLE. Small molecule organic semiconductors on the move: promises for future solar energy technology. Angewandte Chemie-International Edition, 51, 2020-2067(2012).
[11] P DUTTA, W YANG et al. Development of naphtho[1,2-b:5,6-b']dithiophene based novel small molecules for efficient bulk-heterojunction organic solar cells. Chemical Communications, 48, 573-575(2012).
[12] Y A DUAN, Y GENG, H B LI et al. Theoretical characterization and design of small molecule donor material containing naphthodithiophene central unit for efficient organic solar cells. Journal of Computational Chemistry, 34, 1611-1619(2013).
[13] D FERNANDEZ, A VITERISI, V CHALLURI et al. Understanding the limiting factors of solvent-annealed small-molecule bulk-heterojunction organic solar cells from a chemical perspective. ChemSusChem, 10, 3118-3134(2017).
[14] H LI, Y ZHAO, J FANG et al. Improve the performance of the all-small-molecule nonfullerene organic solar cells through enhancing the crystallinity of acceptors. Advanced Energy Materials, 8, 1702377(2018).
[15] Y SHI, C YANG, H LI et al. A-π-D-π-A small-molecule donors with different end alkyl chains obtain different morphologies in organic solar cells. Chinese Chemical Letters, 30, 906-910(2019).
[16] S J LOU, S LOSER, K A LUCK et al. Charge generation mechanism tuned via film morphology in small molecule bulk-heterojunction photovoltaic materials. Journal of Materials Chemistry C, 8, 15234-15252(2020).
[17] Z WEI, K LU, R ZHOU et al. Research progress of small molecule donors with high crystallinity in all small molecule organic solar cells. Acta Chimica Sinica, 79, 284-302(2021).
[18] Y CUI, H YAO, L HONG et al. Achieving over 15% efficiency in organic photovoltaic cells via copolymer design. Advanced Materials, 31, 1808356(2019).
[19] S Q ZHANG, Y P QIN, J ZHU et al. Over 14% efficiency in polymer solar cells enabled by a chlorinated polymer donor. Advanced Materials, 30, 1800868(2018).
[20] Y CUI, H YAO, J ZHANG et al. Over 16% efficiency organic photovoltaic cells enabled by a chlorinated acceptor with increased open-circuit voltages. Nature Communications, 10, 2515(2019).
[21] H X JIANG, X M LI, H WANG et al. Appropriate molecular interaction enabling perfect balance between induced crystallinity and phase separation for efficient photovoltaic blends. ACS Applied Materials & Interfaces, 12, 26286-26292(2020).
[22] H LI, Q WU, R ZHOU et al. Liquid-crystalline small molecules for nonfullerene solar cells with high fill factors and power conversion efficiencies. Advanced Energy Materials, 9, 1803175(2019).
[23] W NI, M M LI, B KAN et al. Fullerene-free small molecule organic solar cells with a high open circuit voltage of 1.15 V. Chemical Communications, 52, 465-468(2016).
[24] W WANG, B CHEN, X JIAO et al. A new small molecule donor for efficient and stable all small molecule organic solar cells. Organic Electronics, 70, 78-85(2019).
[25] H ZHANG, C WANG, X LI et al. The effect of processing conditions on performance of small-molecule organic solar cells. Solar Energy, 157, 71-80(2017).
[26] Y L WANG, Y WANG, L ZHU et al. A novel wide-bandgap small molecule donor for high efficiency all-small-molecule organic solar cells with small non-radiative energy losses. Energy & Environmental Science, 13, 1309-1317(2020).
[27] J GE, L HONG, W SONG et al. Solvent annealing enables 15.39% efficiency all-small-molecule solar cells through improved molecule interconnection and reduced non-radiative loss. Advanced Energy Materials, 2100800(2021).
[28] S LOSER, C J BRUNS, H MIYAUCHI et al. A naphthodithiophene-diketopyrrolopyrrole donor molecule for efficient solution-processed solar cells. Journal of the American Chemical Society, 133, 8142-8145(2011).
[29] S SHINAMURA, I OSAKA, E MIYAZAKI et al. Linear- and angular-shaped naphthodithiophenes: selective synthesis, properties, and application to organic field-effect transistors. Journal of the American Chemical Society, 133, 5024-5035(2011).
[30] K TAKIMIYA, I OSAKA. Naphthodithiophenes: emerging building blocks for organic electronics. Chemical Record, 15, 175-188(2015).
[31] X W ZHU, K LU, H LI et al. Naphthodithiophene-based donor materials for solution processed organic solar cells. Chinese Chemical Letters, 27, 1271-1276(2016).
[32] Y CHANG, Y CHANG, X ZHU et al. Constructing high-performance all-small-molecule ternary solar cells with the same third component but different mechanisms for fullerene and non-fullerene systems. Advanced Energy Materials, 9, 1900190(2019).
[33] C XIA, H WU, H FAN et al. Efficient NDT small molecule solar cells with high fill factor using pendant group engineering. Journal of Materials Chemistry C, 8, 7561-7566(2020).
[34] STOKKUM I H MVAN, D S LARSEN, GRONDELLE RVAN. Global and target analysis of time-resolved spectra. Biochimica Et Biophysica Acta-Bioenergetics, 1657, 82-104(2004).
[35] C HIPPIUS, STOKKUM I H MVAN, E ZANGRANDO et al. Excited state interactions in calix 4 arene-perylene bisimide dye conjugates: Global and target analysis of supramolecular building blocks. Journal of Physical Chemistry C, 111, 13988-13996(2007).
[36] X WANG, B KAN, Z KUANG et al. Unveiling the molecular symmetry dependence of exciton dissociation processes in small-molecular heterojunctions. Journal of Physical Chemistry C, 122, 26851-26856(2018).
[37] C RUCKEBUSCH, M SLIWA, P PERNOT et al. Comprehensive data analysis of femtosecond transient absorption spectra: A review. Journal of Photochemistry and Photobiology C-Photochemistry Reviews, 13, 1-27(2012).
[38] R BERERA, GRONDELLE RVAN, J T M KENNIS. Ultrafast transient absorption spectroscopy: principles and application to photosynthetic systems. Photosynthesis Research, 101, 105-118(2009).
[39] S GELINAS, A RAO, A KUMAR et al. Ultrafast long-range charge separation in organic semiconductor photovoltaic diodes. Science, 343, 512-516(2014).
[40] A C JAKOWETZ, M L BOEHM, A SADHANALA et al. Visualizing excitations at buried heterojunctions in organic semiconductor blends. Nature Materials, 16, 551-557(2017).
[41] M LI, F LIU, X WAN et al. Subtle balance between length scale of phase separation and domain purification in small-molecule bulk-heterojunction blends under solvent vapor treatment. Advanced Materials, 27, 6296-6302(2015).
[42] K SUN, Z XIAO, E HANSSEN et al. The role of solvent vapor annealing in highly efficient air-processed small molecule solar cells. Journal of Materials Chemistry A, 2, 9048-9054(2014).
[43] L XIE, C YANG, R ZHOU et al. Ternary organic solar cells based on two non-fullerene acceptors with complimentary absorption and balanced crystallinity. Chinese Journal of Chemistry, 38, 935-940(2020).
[44] B YANG, Y YUAN, J HUANG. Reduced bimolecular charge recombination loss in thermally annealed bilayer heterojunction photovoltaic devices with large external quantum efficiency and fill Factor. Journal of Physical Chemistry C, 118, 5196-5202(2014).
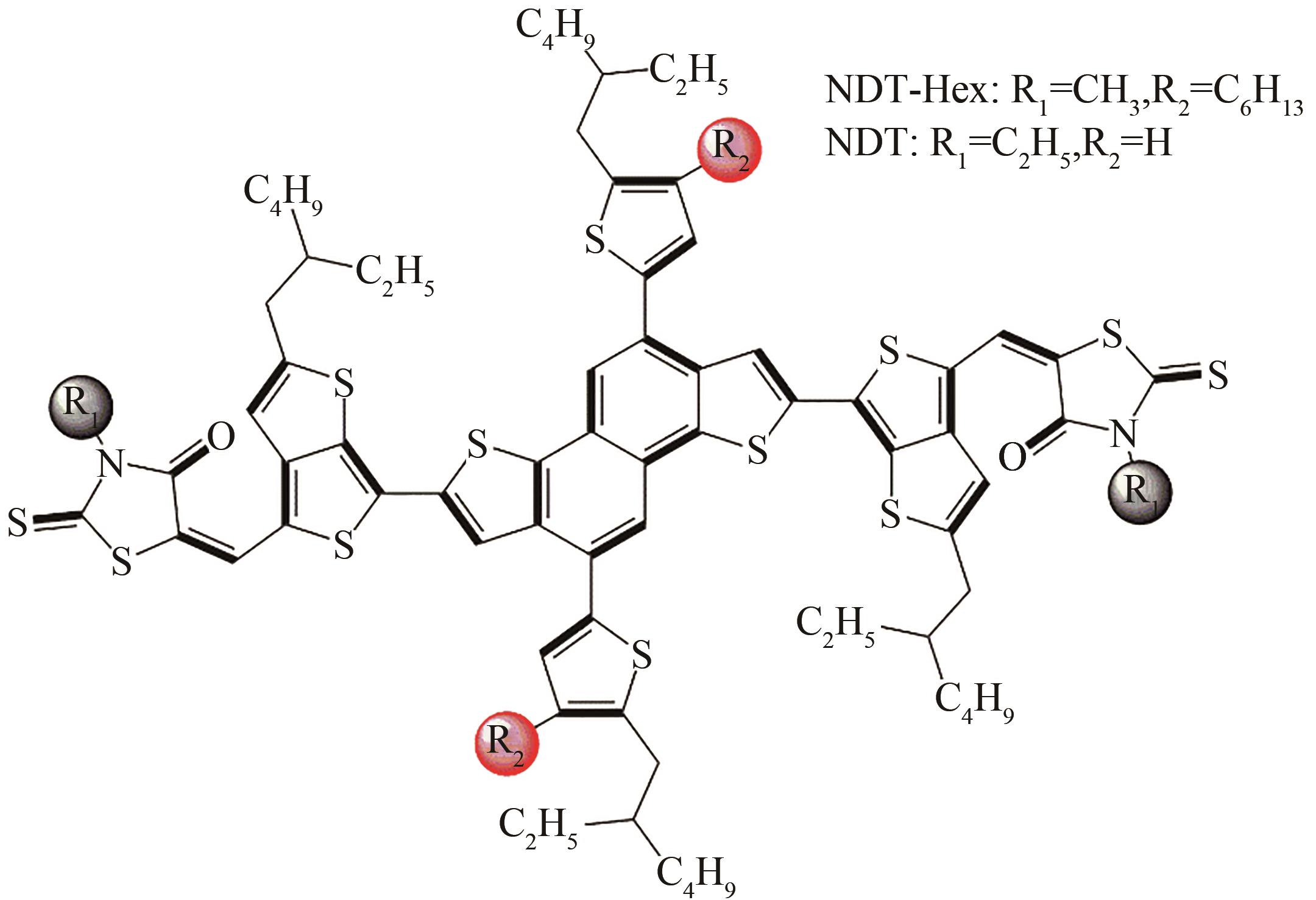
Set citation alerts for the article
Please enter your email address