Abstract
We report 20 Gb/s transmission of four-level pulse amplitude modulation (PAM) signal using a directly modulated tunable distributed Bragg reflector (DBR) laser. Transmission distance over 20 km was achieved without using optical amplifiers and optical dispersion compensation modules. A wavelength tuning range of 11.5 nm and a 3 dB bandwidth greater than 10 GHz over the entire wavelength tuning range were obtained.The wavelength division multiplexed passive optical network (WDM-PON) technology can effectively exploit the capacity of optical fibers and reduce the costs of maintenance, which will be an excellent candidate for 5G front-haul networks[1–4]. As a key technology, a colorless optical network unit (ONU) in WDM-PON systems utilizes tunable lasers to realize high speed and low cost. The wavelength tunable lasers can replace multiple lasers or multi-wavelength laser arrays in traditional transmitters and reduce the costs of production and maintenance. Typical tunable lasers include a wavelength tunable distributed Bragg reflector (DBR) laser[5,6], surface micromachined tunable vertical-cavity surface-emitting laser (VCSEL)[7], V-coupled-cavity laser[8], sampled grating DBR (SG-DBR) laser[9], digital supermode DBR (DS-DBR) laser[10], tunable external-cavity laser (ECL)[11], etc. In terms of simplicity in manufacture and cost, the tunable DBR laser is an ideal choice for WDM-PON systems[12].
Usually, the DBR laser is modulated by non-return-to-zero (NRZ) on–off key (OOK) codes, transmitting one bit per symbol. With the approach of the 200G and 400G era, four-level pulse amplitude modulation (PAM-4) has attracted much attention from both academia and industry[13,14] due to its ability to double the data rate without increasing the required overall bandwidth over conventional NRZ. In the direct modulation schemes, most researches focused on the PAM-4 of a directly modulated distributed feedback (DFB) laser, very few references reported the PAM-4 modulation of DBR lasers. In a previous work reported by Huawei Co., Ltd.[15], 10 Gb/s PAM-4 direct modulation of a DBR laser was demonstrated. In the experiment, the DBR laser could be tuned up to 10 nm with a modulation speed of 5 GBaud using duobinary and PAM-4 modulations; a 40 km reach was achieved using this scheme. The experiment reveals the possibility of combining the PAM-4 direct modulation and wavelength tunable laser technology in a low cost manner.
In this work, we demonstrate PAM-4 modulation and the transmission performance of a three-section tunable DBR laser at a baud rate of 10 GBaud. The DBR laser achieves a continuous wavelength tuning range of 11.5 nm with a modulation bit rate of 20 Gb/s (10 GBaud) using PAM-4 modulation. The PAM-4 signal was transmitted over a 20 km standard single mode fiber (SMF) with a clear, opened eye diagram.
Sign up for Chinese Optics Letters TOC. Get the latest issue of Chinese Optics Letters delivered right to you!Sign up now
The DBR laser consists of three sections, a DBR section, a phase section, and a gain section, as shown in Fig. 1. The active region was InGaAlAs multiple quantum wells (MQWs) to increase its differential gain and reduce the temperature dependence. Polyimide was buried under the electrode pad to decrease the parasitic capacitance. The DBR laser was fabricated as follows. The active region consists of six compressively strained InGaAlAs MQWs and seven InGaAlAs barrier layers, which were sandwiched between two separate confinement heterostructure (SCH) InGaAlAs layers. An InGaAsP layer was butt-jointed with the active layer. After a grating was formed in the DBR section by holographic lithography, a -type InP cladding layer and an InGaAs contact layer were grown. A 3-μm-wide ridge waveguide was fabricated by wet etching. The lengths of the gain, phase, and DBR sections were 300, 100, and 150 μm, respectively. Finally, the devices were cleaved and mounted on an AlN heat sink for measurement. A detailed fabrication process can be found in our previous work[5]. The schematic view of the DBR laser is shown in Fig. 1.
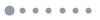
Figure 1.Schematic view of the tunable DBR laser.
The InGaAlAs DBR laser was pigtail packaged, as shown in Fig. 2. The typical light-current characteristic of the packaged InGaAlAs DBR laser is shown in Fig. 3. The threshold current of the device was 22 mA, and the output power was 7.5 mW at the 100 mA driving current when there were no currents injected into the phase and DBR sections.
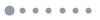
Figure 2.Optical image of the packaged laser.
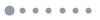
Figure 3.Light-current characteristic of the InGaAlAs DBR lasers at 25°C.
The small signal modulation characteristics are shown in Fig. 4. During the measurement, the gain current was fixed at 100 mA when the phase and DBR current was 0 mA at 25°C. The measured 3 dB modulation bandwidth of the laser was about 12 GHz.
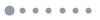
Figure 4.S21 curve of the InGaAlAs DBR laser at 25°C when the gain section, phase section, and DBR section were biased at 100, 0, and 0 mA, respectively.
Figure. 5 shows the wavelength tuning characteristic as a function of the DBR-section current and the side mode suppression ratio (SMSR) of the laser during the tuning of the laser at 25°C. As can be seen, the lasing wavelength can be tuned up to 11.5 nm from 1559.8 to 1548.3 nm as the DBR current increases from 0 to 60 mA. When the DBR current was larger than 60 mA, the thermal effect will dominate the change of refractive index, and the wavelength tuning range cannot be further increased. The SMSR is greater than 35 dB during the entire tuning range, except in the regions where a mode jump occurs. The overlapped optical spectra obtained from the InGaAlAs laser at 25°C are shown in Fig. 6. During the measurement, the resolution of the spectrum analyzer was set to be 0.01 nm.
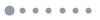
Figure 5.Wavelength tuning properties of InGaAlAs DBR lasers at 25°C.
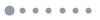
Figure 6.Typical optical spectra obtained from the InGaAlAs DBR lasers at 25°C.
The DBR laser was then modulated by a PAM-4 signal and sent into a coil of SMF (G652D), as shown in Fig. 7. Two channels of NRZ signals with a word length of generated from a pattern generator (SHF-12125B) and then multiplexed to PAM-4 signal by a 3 bit digital to analog converter (DAC, SHF-DAC-613). The PAM-4 signal was combined with the DC driving current by a bias tee and applied on the gain section of the laser. The wavelength was tuned by a current . A digital sampling oscilloscope (Keysight 86100D) was used to observe the eye diagram.
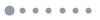
Figure 7.Experimental setup used to measure the PAM-4 transmission characteristics of the tunable DBR laser.
Figure 8 shows the 10 GBaud (20 Gb/s) PAM-4 eye diagram of the tunable DBR laser in the cases of back-to-back (BTB), 10 km, and 20 km transmission when the wavelength was tuned at 1559.8, 1555.7, 1552.4, and 1548.3 nm, respectively. The gain current and the peak-to-peak modulation voltage were set to be 70 mA and 2.1 V, respectively. After transmission of 10 km, the PAM-4 signal is clearly opened, and, after 20 km transmission, the eye is still distinguishable.
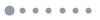
Figure 8.PAM-4 eye diagrams of tunable DBR laser for BTB, 10 km, and 20 km transmission when the tuned wavelength is (a) 1559.8 nm, (b) 1555.7 nm, (c) 1552.4 nm, and (d) 1548.3 nm.
The bit error ratio (BER) of the directly modulated DBR laser was evaluated by off-line processing. Figure 9 shows the obtained BER as a function of received optical power after different distances of transmissions when the tuned wavelength is 1559.8 nm. The corresponding DBR currents are 0 mA. Because the real-time signal is deteriorating and the amount of data is not so large as when the BER is less than , the minimum value of the BER is set to in the calculation procedure.
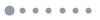
Figure 9.20 Gb/s PAM-4 BER performance of tunable DBR laser for BTB, 10 km, and 20 km transmission when the tuned wavelength is 1559.8 nm.
In summary, a three-section InGaAlAs/InP DBR laser was fabricated with a continuous wavelength tuning range of 11.5 nm and a 3 dB bandwidth greater than 11 GHz. Transmission of a 20 Gb/s PAM-4 signal over 20 km is achieved in the C band. The direct PAM-4 modulation of the DBR laser provides a prospective solution to 5G front-haul WDM-PON systems.
References
[1] P. P. Baveja, M. Li, D. Wang, C. Hsieh, H. Zhang, N. Ma, Y. Wang, J. Lii, Y. Liang, C. Wang, I. L. Ho, J. Zheng. Optical Fiber Communications Conference and Exhibition, Th4G.6(2017).
[2] A. Samani, D. Patel, M. Chagnon, E. El-Fiky, R. Li, M. Jaques, N. Abbadía, V. Veerasubramanian, D. V. Plant. Opt. Express, 25, 13252(2017).
[3] C. Sun, S. H. Bae, H. Kim. IEEE Photon. Technol. Lett., 29, 130(2017).
[4] Q. Zhang, N. Stojanovic, C. Prodaniuc, C. Xie, M. Koenigsmann, P. Laskowski. Opt. Lett., 41, 4449(2016).
[5] D. Zhou, S. Liang, L. Zhao, H. Zhu, W. Wang. Opt. Express, 25, 2341(2017).
[6] L. Yu, H. Wang, D. Lu, S. Liang, C. Zhang, B. Pan, L. Zhang, L. Zhao. IEEE Photon. J., 6, 1501308(2014).
[7] C. Gierl, T. Gruendl, P. Debernardi, K. Zogal, C. Grasse, P. Meißner, S. Jatta, F. Küppers. Opt. Express, 19, 17336(2011).
[8] J. Jin, L. Wang, T. Yu, Y. Wang, J. He. Opt. Lett., 36, 4230(2011).
[9] T. Suzuki, H. Arimoto, T. Kitatani, A. Takei, T. Taniguchi, K. Shinoda, J. Igarashi, A. Nakamura, K. Naoe, M. Okayasu, S. Tanaka, S. Tsuji. IEEE Photon. Technol. Lett., 23, 1391(2011).
[10] A. J. Ward, G. Busico, N. D. Whitbread, L. Ponnampalam, J. P. Duck, D. J. Robbins. IEEE J. Quantum Electron., 42, 1122(2006).
[11] J. H. Lee, M. Y. Park, C. Y. Kim, S. Cho, W. Lee, G. Jeong. IEEE Photon. Technol. Lett., 17, 1956(2005).
[12] J. L. Wei, K. Grobe, C. Sanchez, E. Giacoumidis, H. Griesser. Opt. Express, 23, 28271(2015).
[13] K. Zhong, X. Zhou, T. Gui, L. Tao, Y. Gao, W. Chen, J. Man, L. Zeng, A. P. T. Lau, C. Lu. Opt. Express, 23, 1176(2015).
[14] J. Shi, J. Zhang, N. Chi, J. Yu. Opt. Express, 25, 32254(2017).
[15] N. Cheng, X. Yan, N. Chand, F. Effenberger. Asia Communications and Photonics Conference, ATh3E.4(2013).