
- Chinese Optics Letters
- Vol. 22, Issue 3, 033801 (2024)
Abstract
1. Introduction
Liquid crystals (LCs) represent intermediate states between solids and liquids, exhibiting both crystal-like order and liquid fluidity. The distribution of the director and optical properties strongly depend on surface properties and temperature. LCs demonstrate high sensitivity to external fields and exhibit a broad range of dielectric anisotropies, allowing them to respond across a wide frequency range, from ultraviolet to microwave wavelengths[1]. Research in this field has been steadily advancing beyond information display applications and venturing into the exciting realm of photonics, demonstrating immense scientific potential[2].
LCs possessing fluorescence emission capabilities are referred to as luminescent LCs (LLCs). These materials exhibit LC properties with excellent luminescent characteristics[3], including linearly polarized emission[4] and circularly polarized luminescence[5], and are widely applied in fields such as optical information display[6]. LLCs can be created by doping them with fluorescent materials, such as fluorescent dyes[7], rare earth elements[8], and quantum dots[9]. Doping alters the optical properties of LCs, enabling them to emit fluorescent light. However, when fluorescent materials are introduced to form LLCs, they may interact with LC molecules, leading to fluorescence quenching, which reduces the fluorescence emission intensity. This limits the fluorescence performance of LCs and affects their applications. To address the issue of fluorescence quenching, in 2001, Tang et al. proposed aggregation-induced emission (AIE) in siloles[10]. LLCs can also be obtained by synthesizing LC-conjugated polymers, which offer advantages over previous approaches, including improved stability, a more uniform distribution of luminescent units[11], and facile processing[12]. Liu et al. constructed LLC molecules with efficient solid-state luminescence by chemically conjugating tetraphenylene cores with aggregation-induced luminescence and luminescent mesogenic tolane moieties[13]. Zhang et al. synthesized the first columnar rufigallol LC with AIE groups, demonstrating good fluorescence properties in an aggregated state[14]. The fluorescent properties of LLCs strongly depend on their molecular structure[15]. Yu et al. identified the fluorescence emission source as the biphenyl group in the polymer side chain, with the fluorescence intensity decreasing as the spacer length decreases[16]. Moreover, Tang et al. constructed fluorescence patterns based on LC copolymers using nanoimprint lithography[17]. Zhao et al. successfully achieved photopatterns in a luminescent LC device via photoalignment technology[18].
The process described earlier typically involves generating fluorescence in LCs via UV irradiation, resulting in down-conversion luminescence. In this process, the emitted wavelength is longer and has weaker photon energy than the input photon energy, following the principle of Stokes’ rule. Another phenomenon, known as up-conversion luminescence, deviates from Stokes’ rule and offers several advantages, including multicolor emission, strong penetration, a high signal-to-noise ratio, and multiphoton excitation[19]. Achieving up-conversion luminescence in LCs typically involves incorporating fluorescent materials via doping. For example, Guo et al. co-doped a chiral fluorescent photoswitch and up-conversion nanoparticles (UCNPs) into a nematic LC (NLC) medium to investigate the effects of up-conversion luminescence on reflective color switching and fluorescence tuning in cholesteric LC (CLC) cells[20].
Sign up for Chinese Optics Letters TOC. Get the latest issue of Chinese Optics Letters delivered right to you!Sign up now
Femtosecond-laser-induced materials generate two-photon absorption (2PA), resulting in up-conversion fluorescence. This advanced technology offers several advantages, such as high spatial selectivity, reduced photodamage, and enhanced sensitivity[21]. Therefore, studying the interaction between light and matter is crucial[22]. This process holds vast potential for applications in three-dimensional optical data storage[23] and two-photon-excited fluorescence microscopy[24]. Qian et al. measured the fluorescence spectra of 2PA and up-conversion fluorescence in symmetrical compounds using an 800-nm femtosecond laser. Furthermore, they studied the solvatochromic effect of these compounds in various solvents[25]. Vivas et al. examined the influence of aluminum content in AlxGa1-xN thin films and the n-type doping concentration in GaN thin films on their 2PA coefficients by analyzing femtosecond-level 2PA spectra[26]. In their study on polycrystalline zinc selenide (ZnSe), excited by 775 nm, 1 kHz femtosecond laser pulses, Li et al. observed that the two-photon-induced fluorescence exhibited blue fluorescence and self-absorption near the band edge[27]. To date, femtosecond-laser-induced LCs generating up-conversion fluorescence and forming microstructured fluorescence have not been reported.
The introduction of fluorescent components into LCs and construction of LCP can complicate the pretreatment process, potentially limiting practical application of these materials. This study investigated the use of pure NLC material NJU-LDn-4 for direct fluorescence emission generated by a femtosecond laser, eliminating the need for additional fluorescent doping. Fluorescence was generated via the 2PA mechanism, and specific fluorescent microstructures were achieved using photoalignment technology. This approach enables the preparation of high-resolution luminescent two-dimensional patterns.
2. Experiment
The experimental setup for LC fluorescence generation using femtosecond laser excitation is shown in Fig. 1(a). The pump source used was a titanium sapphire femtosecond laser with a center wavelength of 800 nm, pulse width of 100 fs, and repetition rate of 1 kHz. The laser beam was incident parallel to the LC cell. Fluorescence spectra were measured using a spectrometer PG 2000 Pro. Meanwhile, a fluorescent beam with graphic information on the LC cell was monitored and recorded in real-time using a CCD camera, and the laser beam was loosely focused on the LC cell with a spot diameter of approximately 2 mm. The LC cell comprised two parallel fused silica substrates separated by 250-µm thick Mylar films and infiltrated with the LC NJU-LDn-4. Both substrates were spin-coated with a 0.5% (mass fraction) solution of sulfonic azo dye (SD1), which served as an alignment layer. The NJU-LDn-4 hybrid material mainly comprises derivatives with fluorinated biphenyltolanes[28], as illustrated in the inset. The presence of carbon–carbon triple bonds ensures a strong conjugation effect, and the biphenyl group is considered to be the main component of the fluorescence emission[16,29–31]. The experimental fluorescence of the sample is shown in Fig. 1(b).
Figure 1.(a) Schematic of the experimental setup for femtosecond laser-induced fluorescence up-conversion in LCs. The inset shows the primary components of NJU-LDn-4. (b) The experimental fluorescence of the sample.
3. Results and Discussion
3.1. Spectral measurement of the LC
The absorption and transmittance spectra of NJU-LDn-4 are shown in Figs. 2(a) and 2(b), respectively. The absorption intensity is highest at 400 nm, rapidly decreasing between 400 and 500 nm and then gradually slowing down beyond 500 nm. No absorption peak can be observed at 800 nm, and the absorption intensity is relatively low. The transmittance reaches its minimum at 400 nm and increases with increasing wavelengths. Figures 2(c) and 2(d) show the excitation and emission spectra, with central wavelengths of 390 nm and 450 nm, respectively, resulting in a Stokes shift of about 60 nm.
Figure 2.(a) Absorption and (b) transmittance spectra of the NJU-LDn-4. (c) Excitation and (d) emission spectra of NJU-LDn-4.
3.2. Comparison of the effects of femtosecond and continuous lasers on fluorescence
Continuous lasers with a wavelength of 405 nm were used to excite the LC and were compared to femtosecond laser excitation at 800 nm. A continuous purple laser was applied to the LC cell sample, and the detected spectrum is shown in Fig. 3(a). The LC exhibited fluorescence emission when excited by the purple laser with a central wavelength of 492 nm, resulting in blue fluorescence emission. The Stokes shift was 87 nm and a fluorescence image was captured under UV irradiation at 405 nm. The spectrum resulting from excitation of the LC using a femtosecond laser pulse is depicted in Fig. 3(b). Compared with UV-pumped single-photon fluorescence, it exhibits a similar fluorescence spectrum with a 9-nm shift in the central wavelength. The fluorescence image maintained its blue color. In contrast, employing a continuous laser operating at 808 nm to excite the LC resulted in the absence of fluorescence emission (not illustrated here). However, when stimulated by a femtosecond laser with a wavelength of 800 nm, the NJU-LDn-4 generated up-conversion fluorescence with a central wavelength of 501 nm. Consequently, a preliminary conclusion can be drawn: femtosecond laser excitation of NJU-LDn-4 involves two-photon absorption fluorescence.
Figure 3.(a) Fluorescence spectrum of the LC material excited by a 405-nm continuous laser. The inset shows the fluorescence generated by the continuous laser-induced LC cell. (b) Fluorescence spectrum of the LC material excited by an 800-nm femtosecond laser. The inset shows the fluorescence produced by the femtosecond laser-induced LC cell.
The peak positions of the single- and two-photon fluorescence showed no significant changes, and strong fluorescence was visible to the naked eye. However, the obtained fluorescence image using the femtosecond laser exhibits a distinct concentric ring structure [inset of Fig. 3(b)]. Compared with the single-photon fluorescence generated by continuous laser excitation, the femtosecond laser confines the fluorescence to its focal point, and longer wavelengths are significantly less affected by scattering. Furthermore, two-photon fluorescence generated by the femtosecond laser offers a higher resolution. Under focused laser conditions, the 2PA effect can only be observed within a cubic region near the focal point corresponding to the excitation wavelength, whereas single-photon fluorescence occurs throughout the optical path.
3.3. Two-photon fluorescence characteristics
The fluorescence spectra of NJU-LDn-4 excited by the femtosecond laser at various pump powers are shown in Fig. 4(a). The fluorescence intensity increases gradually with power, experiencing a small enhancement from 0.5 to 1.5 W, followed by a significant enhancement from 1.5 to 3 W. When normalizing the fluorescence intensity at different pump powers, Fig. 4(b) illustrates the relationship between the fluorescence peaks and pump power, with experimental data indicated by black squares. The fluorescence intensity exhibits a clear quadratic growth trend, consistent with the theoretical relationship between the fluorescence intensity (
Figure 4.(a) Fluorescence spectra of NJU-LDn-4 at different pump powers. (b) Quadratic fitting curve of fluorescence peaks under different pump power excitations.
In this case, the relationship follows an equation with
3.4. Microstructured fluorescence
LC photoalignment technology is a non-contact method for aligning LCs using polarized light illumination. Unlike the rubbing alignment method, this approach offers several advantages, including being pollution-free, electrostatic-free, and facilitating multidomain alignment in microregions[33]. The design involves using LC cells with microstructured patterns to investigate fluorescence emission phenomena and analyze fluorescence characteristics.
LC cell structures were designed using a digital micro-mirror device (DMD)-based dynamic microlithography system, which was utilized to perform a multi-step and partially overlapping exposure process to implement photopatterning on SD1, including a periodic grating structure, as shown in Fig. 5(a), along with a corresponding computer-generated hologram[34] featuring a topological charge value of
Figure 5.(a) Periodic grating pattern (100 px represents one cycle) of the LC cell. (b) Fluorescence from the grating LC cell generated by a femtosecond laser and (c) continuous laser excitation. (d) Forked grating pattern with m = 4 and a scale bar of 100 µm. (e) Fluorescence of the forked LC cell generated by a femtosecond laser and (f) continuous laser excitation.
The fluorescence of the LC cells under femtosecond laser and continuous laser induction was investigated. Figures 5(b) and 5(e) show clear fluorescence striped and quadruple patterns generated by the femtosecond laser. Figures 5(c) and 5(f) show the fluorescence under continuous laser UV excitation, with no observable patterns. The microstructure design in the LC cell of the NJU-LDn-4 material directly influenced the fluorescence patterns generated by the femtosecond laser. We believe that under the femtosecond laser excitation, the LC initially produces fluorescence, which is then modulated by the microstructure of the LC, resulting in the observed patterns. Compared with continuous laser induction, femtosecond lasers produce two-photon fluorescence using longer wavelengths, resulting in less damage and deeper penetration. Consequently, the generated fluorescence patterns were clearer. Based on these properties, all types of fluorescence patterns can be generated by designing the LC microstructures.
4. Conclusion
This study presents the phenomenon of fluorescence generated by a femtosecond laser in NLCs. The fluorescence, absorption, and transmission spectra of NJU-LDn-4 were analyzed and compared with single-photon fluorescence generated by continuous laser excitation. The physical mechanism of femtosecond-laser-induced LC fluorescence emission was determined to be 2PA. Photoalignment of LC microstructures produces high-resolution fluorescence patterns under femtosecond laser irradiation, in contrast to continuous UV laser irradiation. Because LCs are excellent electro-optic materials, their fluorescence can be dynamically adjusted by applying voltage. These findings can advance the development of functional fluorescent LCs, especially in creating tunable fluorescence patterns via photoalignment technology. Such advancements may find applications in fluorescence microscopy imaging, advanced optoelectronic devices, optical information processing, and biochemical sensing.
References
[1] H. Maune, M. Jost, R. Reese et al. Microwave liquid crystal technology. Crystals, 8, 355(2018).
[21] O. V. Przhonska, S. Webster, L. A. Padilha et al. Two-photon absorption in near-IR conjugated molecules: design strategy and structure–property relations. Advanced Fluorescence Reporters in Chemistry and Biology I, 105(2010).
[25] Y. Qian, X. Q. Zhu, W. Huang et al. Two-photon absorption and upconversion fluorescence by using a femtosecond Ti:sapphire laser in symmetrical chromophores. J. Funct. Mater., 39, 1774(2008).
[33] V. G. Chigrinov, V. M. Kozenkov, H.-S. Kwok. Photoalignment of Liquid Crystalline Materials: Physics and Applications(2008).
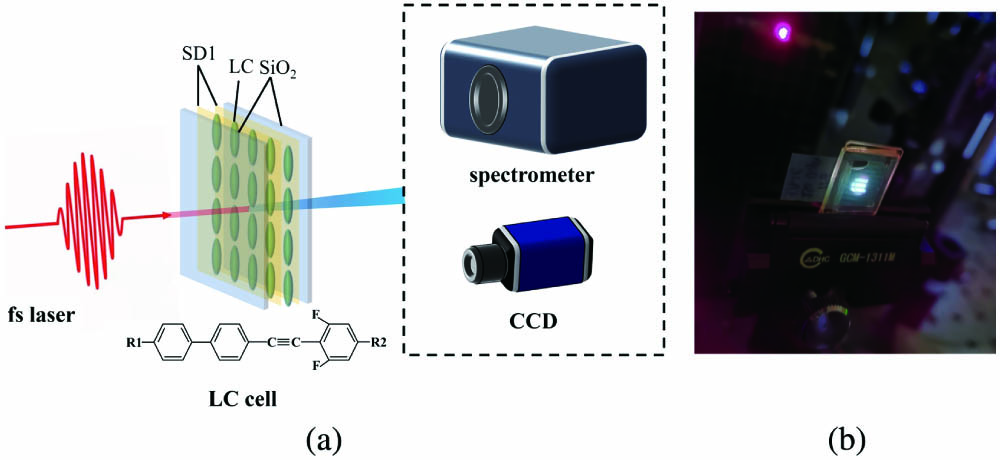
Set citation alerts for the article
Please enter your email address