
Fig. 1. Typical examples of phase-only meta-holography, amplitude-only meta-holography and complex amplitude meta-holography.(
a) 3D on-axis transmission-type phase-only meta-hologram composed of gold nanorod arrays
38. (
b) Phase-only metasurface-based broadband hologram with high tolerance to fabrication errors consisting of an elongated nanoaperture array
40. (
c) Amplitude-only meta-hologram enabled by a random photon sieve
42. (
d) Dielectric metasurface for complete and independent control of the optical amplitude and phase by adjusting the geometrical parameters and orientation angles of meta-atoms. The middle images are scanning electron microscopy (SEM) images of fabricated samples. Experimental reconstruction overlaying the separately measured pictures at 1.65 μm (marked as red color) and 0.94 μm (marked as blue color) wavelengths
48. Figure reproduced with permission from: (a) ref.
38, under a Creative Commons Attribution 3.0 Unported Licence; (b) ref.
40, (d) ref.
48, under a Creative Commons Attribution 4.0 International License; (c) ref.
42, Springer Nature.
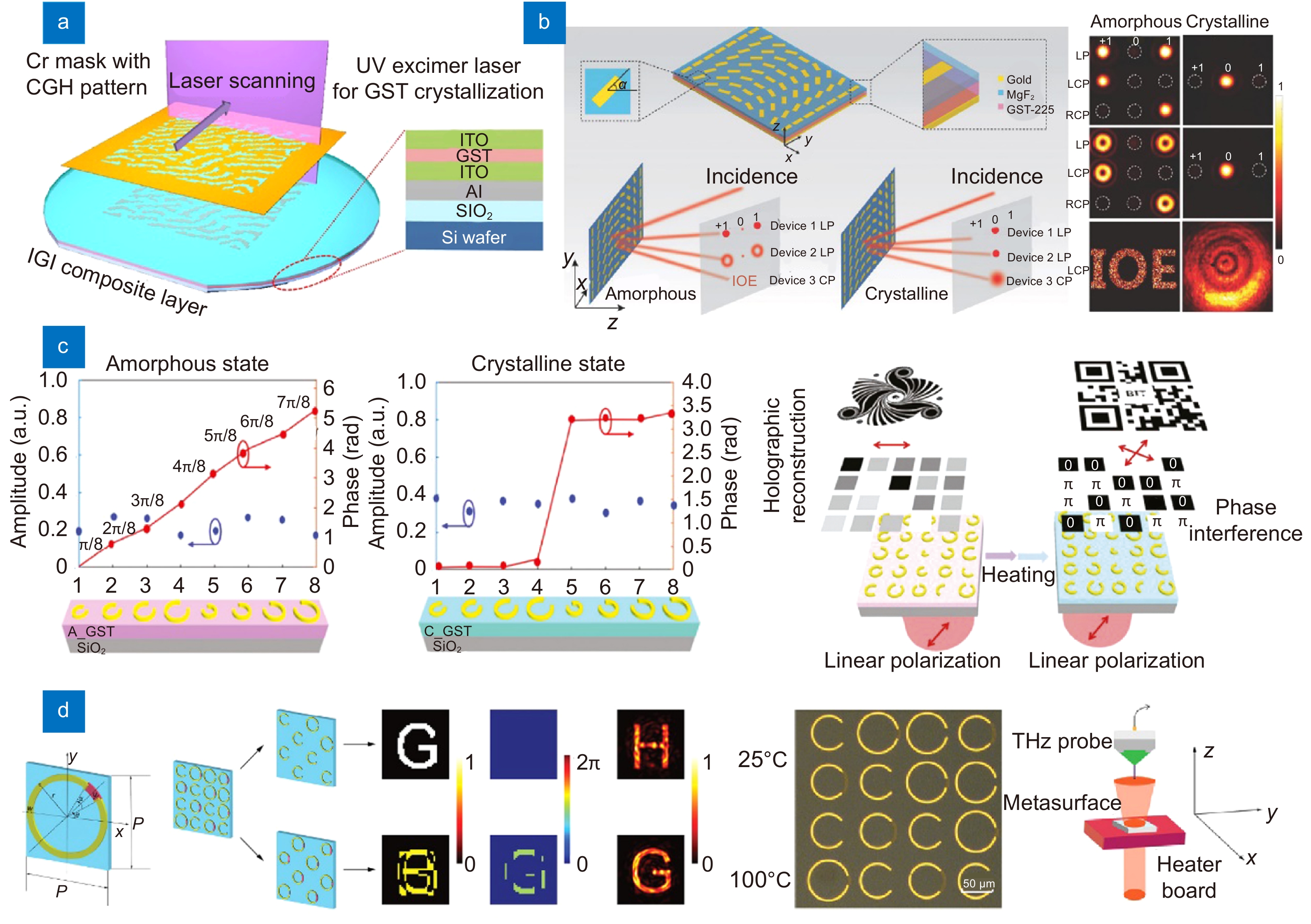
Fig. 2. Tunable metasurface holography based on phase transitions.(
a) Schematic of the proposed multilayered GST-based sample and its hologram recording process. A thin film of a PCM encapsulated by dielectric layers can be used for the hologram panel by means of excimer laser lithography. (
b) Schematic of the demonstrated switchable photonic SOIs. Different optical performances of the three designed metadevices can be switched between when the GST layer is in the amorphous or crystalline state. (
c) Schematic of double functionalities using the simultaneous phase modulation and interference strategy. The continuous phase modulation under the amorphous state can be converted to a binary phase modulation under the crystalline state. (
d) Schematic of thermally dependent dynamic meta-holography using a vanadium dioxide integrated metasurface. The meta-hologram consists of two sets of resonators, which generate certain holographic images with predesigned amplitude and phase distributions. Figure reproduced with permission from: (a) ref.
77, (c) ref.
76, under a Creative Commons Attribution 4.0 International License; (b) ref.
78, under the terms of the Creative Commons Attribution License; (d) ref.
83, John Wiley and Sons.
Fig. 3. Tunable metasurface holography based on chemical reactions. (
a) Chemically active metasurfaces allow independent manipulation of addressable subwavelength pixels at visible frequencies. Plasmonic nanorods tailored to exhibit hierarchical reaction kinetics upon hydrogenation/dehydrogenation constitute addressable pixels in multiplexed metasurfaces. (
b) An iterative hologram algorithm based on the Fidoc method builds up a quantified phase relation, which allows the reconfigurable phase profile to reshape the reconstructed image. Figure reproduced from: (a) ref.
90, AAAS; (b) ref.
89, Optical Society of America.
Fig. 4. Rewritable and stretchable meta-holography methods. (
a) Tunable metasurface hologram composed of functional graphene oxide materials for achieving 3D holography with wide viewing angles, which is rewritable by pumping with femtosecond laser pulses. (
b) Reconfigurable phase-only computer-generated metasurface holograms with up to three image planes operating in the visible regime fabricated with gold nanorods on a stretchable polydimethylsiloxane substrate. Upon stretching, the holographic image displayed by these devices can be switched between multiple distinct images. Figure reproduced with permission from: (a) ref.
71, under a Creative Commons Attribution 4.0 International License. (b) ref.
87, American Chemical Society.
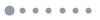
Fig. 5. Wavelength-multiplexed meta-holography.(
a) Schematic of the designed multicolor meta-hologram under linearly polarized illumination. The phase-modulated multicolor meta-hologram enables the formation of polarization-dependent images in three primary colors. (
b) Schematic of visible-frequency silicon metasurfaces formed by three kinds of nanoblocks multiplexed in a subwavelength unit, which are capable of wavefront manipulation for red, green, and blue light simultaneously. (
c) Schematic of full-color plasmonic metasurface holograms with both amplitude and phase modulations for reconstructing 2D and 3D holographic color images. (
d) Schematic of the off-axis illumination method for the full-color meta-hologram. The image on the top right shows the experimental results of a colorful flower image based on plasmonic nanoslit antennas. Figure reproduced with permission from: (a) ref.
99, (b) ref.
100, (c) ref.
103, American Chemical Society. (d) ref.
102, under a Creative Commons Attribution NonCommercial License 4.0.
Fig. 6. Polarization-multiplexed meta-holography. (
a) Schematic diagram and experimental realization of a cartoon dog and cat with tailored Si nanofins for orthogonal circular polarization multiplexing. (
b) Chiral holograms that project different images depending on the handedness of the reference beam by incorporating a geometric phase. (
c) Schematic of a structurally birefringent dielectric metasurface projecting a desired polarization pattern encoding an RGB image with arbitrary complexity. (
d) Schematic of Malus metasurfaces based on a one-to-four mapping and two independent information channels for intensity and phase manipulation. Figure reproduced with permission from: (a) ref.
112, American Physical Society. (b) ref.
113, under a Creative Commons Attribution NonCommercial License 4.0. (c) ref.
115, American Chemical Society. (d) ref.
116, under a Creative Commons Attribution 4.0 International License.
Fig. 7. Angle-multiplexed meta-holography. (
a) Angle-multiplexed metasurfaces composed of reflective high-contrast dielectric U-shaped meta-atoms, whose response under illumination from different angles can be controlled independently. (
b) Schematic illustrations of the coherent pixel polarization meta-holography scheme and angle-multiplexed metasurface. (
c) Schematic illustrations of the imaging information capacity increment by transferring the evanescent wave to the propagating wave through off-axis illumination. Figure reproduced with permission from: (a) ref.
117, under a Creative Commons Attribution 4.0 International License; (b) ref.
118, John Wiley and Sons; (c) ref.
119, Royal Society of Chemistry.
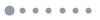
Fig. 8. OAM-multiplexed meta-holography.(
a) Schematic of metasurface orbital angular momentum holography by utilizing the strong orbital angular momentum selectivity offered by meta-holograms consisting of GaN nanopillars with discrete spatial frequency distributions. (
b) Schematic of ultrahigh-dimensional OAM-multiplexed holography based on a large-scale complex-amplitude OAM-multiplexed metasurface hologram. (
c) Schematic of the OAM meta-transformer designed based on three OAM states, which can reconstruct “0”, “3” and “6”. (
d) Schematic of OAM multiplexing in different polarization channels using a birefringent metasurface for holographic encryption. (
e) Schematic of a dynamic metasurface for holographic pattern switching through OAM multiplexing. Switchable vector beams of different polarization states and switchable vortex beams of different topological charges can be implemented through simple hydrogenation and dehydrogenation of the same metasurfaces. Figure reproduced with permission from: (a) ref.
120, (c) ref.
123, (e) ref.
125, under a Creative Commons Attribution 4.0 International license; (b) ref.
122 , Springer Nature; (d) ref.
124, under an ACS AuthorChoice License.
Fig. 9. Space channel-multiplexed meta-holography.(
a) Schematic of a cinematography-inspired metasurface holographic movie. Time-lapse images were reconstructed using sequentially arranged metasurface hologram frames. (
b) Schematic of a space channel multiplexed metasurface. A structured laser beam opens a specific space channel in the designed sequence, and continuous frames of a holographic video are displayed. Figure reproduced with permission from: (a) ref.
126, under the terms of the OSA Open Access Publishing Agreement. (b) ref.
127, under a Creative Commons Attribution License 4.0.
Fig. 10. Nonlinear and reprogrammable meta-holography.(
a) Nonlinear holographic metasurfaces. Linear holography for reconstruction of the letter “X” at infrared wavelengths, and nonlinear holographic images for reconstruction of the letters encoded in different circular polarization states. (
b) Schematic of a reprogrammable metasurface with arbitrary holographic images for optical encryption. Holographic image of the metasurface when it is illuminated with the modulated incident beam and an incorrect uniform laser beam. By playing a set of holographic frames sequentially, a video of the pinball game from start to end can be achieved. Figure reproduced with permisson from: (a) ref.
131, (b) ref.
132, under a Creative Commons Attribution 4.0 International License.
Fig. 11. Multi-dimensions multiplexed meta-holography.(
a) Schematic of (2
6–1) spin- and wavelength-encoded holograms by manipulating six bases of incident photons simultaneously to reconstructed 6-bit wavelength- and spin-dependent multicolor images. (
b) Schematic of a multi-freedom metasurface which achieving full-color complex-amplitude vectorial meta-hologram by combining geometric Pancharatnam-Berry phase and detour phase. Figure reproduced with permission from: (a) ref.
133, American Chemical Society; (b) ref.
134, John Wiley and Sons.