Jintian Lin, Saeed Farajollahi, Zhiwei Fang, Ni Yao, Renhong Gao, Jianglin Guan, Li Deng, Tao Lu, Min Wang, Haisu Zhang, Wei Fang, Lingling Qiao, Ya Cheng, "Electro-optic tuning of a single-frequency ultranarrow linewidth microdisk laser," Adv. Photon. 4, 036001 (2022)

Search by keywords or author
- Advanced Photonics
- Vol. 4, Issue 3, 036001 (2022)
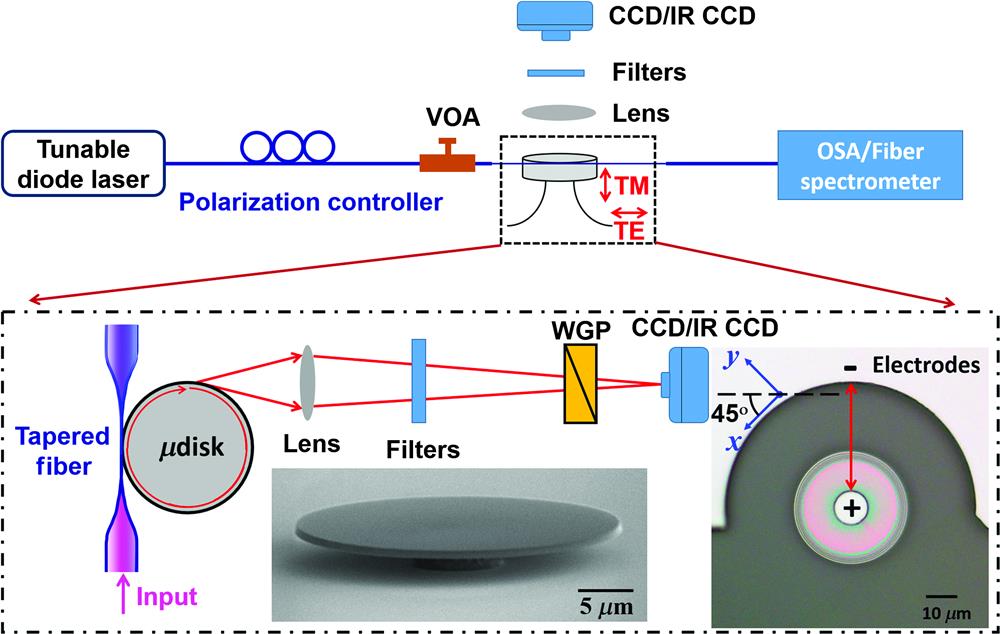
Fig. 1. The experimental setup for lasing and polarization measurement. A variable optical attenuator (VOA) was used to tune the input power into the -cut microdisk. TM and TE represent transverse magnetic and transverse electric polarization states, respectively. Inset: SEM image of the microdisk (without electrodes) and optical micrograph of the microdisk integrated with Cr electrodes.
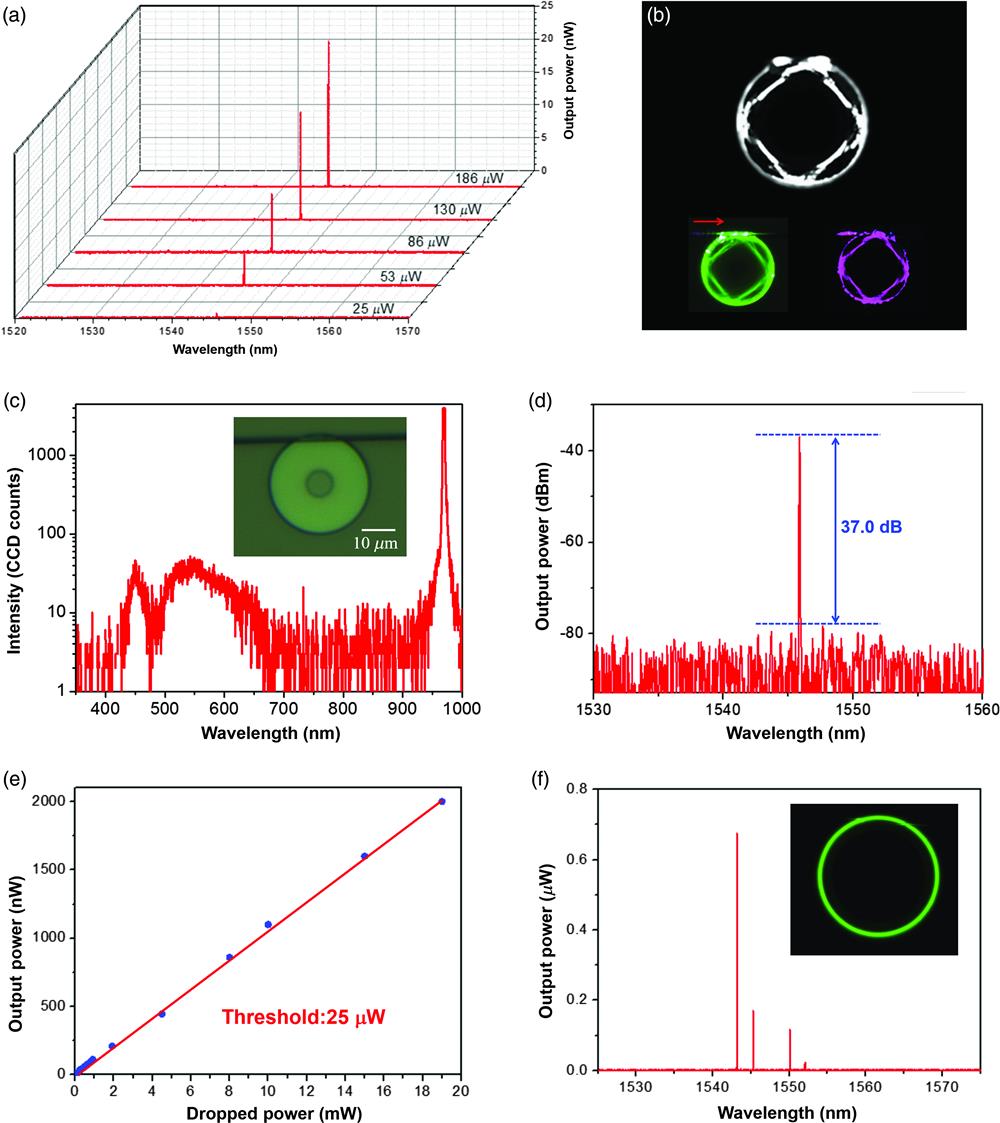
Fig. 2. (a) Spectra of the microlaser output powers at different pump power levels. (b) The optical micrograph of the square lasing mode at 1546-nm wavelength. Inset: the optical micrographs of the square modes of the upconversion fluorescence around the 550-nm wavelength (left) and the pump light (right). (c) Spectrum of the upconversion fluorescence and the pump light. Inset: optical micrograph of the tapered fiber coupled with the microdisk. (d) Spectrum of lasing. (e) Laser output power versus pump power dropped to the cavity shows a pump threshold of . (f) Spectrum of multiple-mode lasing when pumping with a WGM at 971.5-nm wavelength. Inset: the optical micrograph of the upconversion fluorescence, showing a circular pattern along the disk periphery.
![Transmission spectra of the tapered fiber coupled with the microdisk. (a) Transmission spectrum around the pump wavelength, where each blue line segment with two arrows indicates an FSR. (b) The Q factor of the pump mode. (c) The measured highest Q factor of the conventional WGM [indicated with a green triangle in (a)] within one FSR. (d) Spectrum around the lasing wavelength. (e) The Q factor of the lasing mode. (f) The measured highest Q factor of the conventional WGM [indicated with a green rhombus in (d)] within one FSR.](/Images/icon/loading.gif)
Fig. 3. Transmission spectra of the tapered fiber coupled with the microdisk. (a) Transmission spectrum around the pump wavelength, where each blue line segment with two arrows indicates an FSR. (b) The factor of the pump mode. (c) The measured highest factor of the conventional WGM [indicated with a green triangle in (a)] within one FSR. (d) Spectrum around the lasing wavelength. (e) The factor of the lasing mode. (f) The measured highest factor of the conventional WGM [indicated with a green rhombus in (d)] within one FSR.
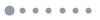
Fig. 4. Linewidth measurement. (a) The experimental setup. (b) The spectrum of the detected beating signal for an output power of . (c) The measured frequency noise. (d) The linewidth is a function of the output power of the microlaser.
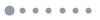
Fig. 5. (a) Lasing wavelength drift with different pump powers. (b) Lasing wavelength versus pump power. The linear fitting (red curve) shows a slope of . Distributions of (c) the scalar electric potential and (d) the vectorial electric field in the microdisk. The arrows denoting the vectorial electric field have a length proportional to the logarithm of the electric field magnitude. The material layers from top to bottom were Cr, LN, silica, and LN, respectively. (e) Lasing wavelength drift with the applied voltage. (f) Lasing wavelength versus applied voltage, showing a high tuning efficiency of .
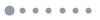
Fig. 6. The intensity distributions of (a) the square mode and (b) the fundamental WGM at the pump wavelengths. Left column: (c) the square mode, (f) the fundamental WGM, and (i) high order WGM at lasing wavelengths. (d)–(k) The intensity distributions of the integrand between each laser mode in the left column and either pump mode in the top row. Here, the larger overlap ( ) in (k) between the pump fundamental WGM and laser high-order WGM suggests that both the fundamental and high-order WGMs are likely to simultaneously lase, while in the case of being pumped by a square mode, the high-order WGM will not lase due to a lower overlap ( ).
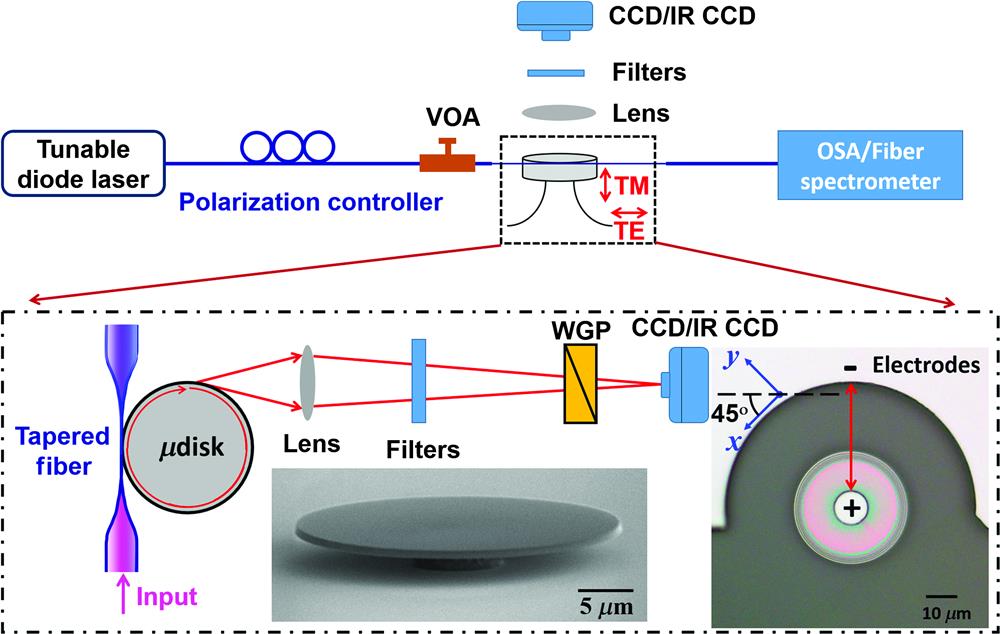
Set citation alerts for the article
Please enter your email address