B. King, T. Heinzl, "Measuring vacuum polarization with high-power lasers," High Power Laser Sci. Eng. 4, 010000e5 (2016)

Search by keywords or author
- High Power Laser Science and Engineering
- Vol. 4, Issue 1, 010000e5 (2016)
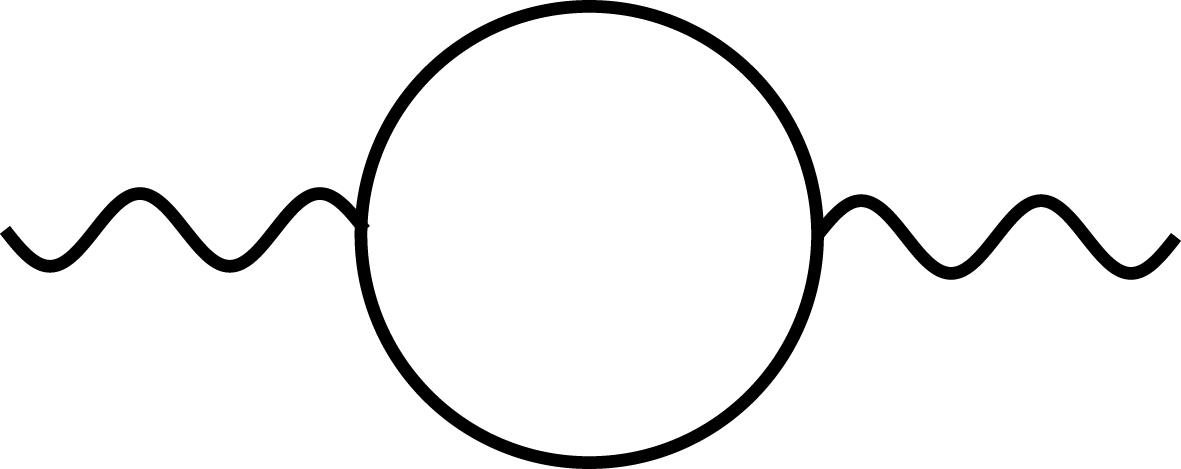
Fig. 1. Vacuum polarization loop in QED. Wavy and straight lines represent photons and fermions (electrons and positrons), respectively.
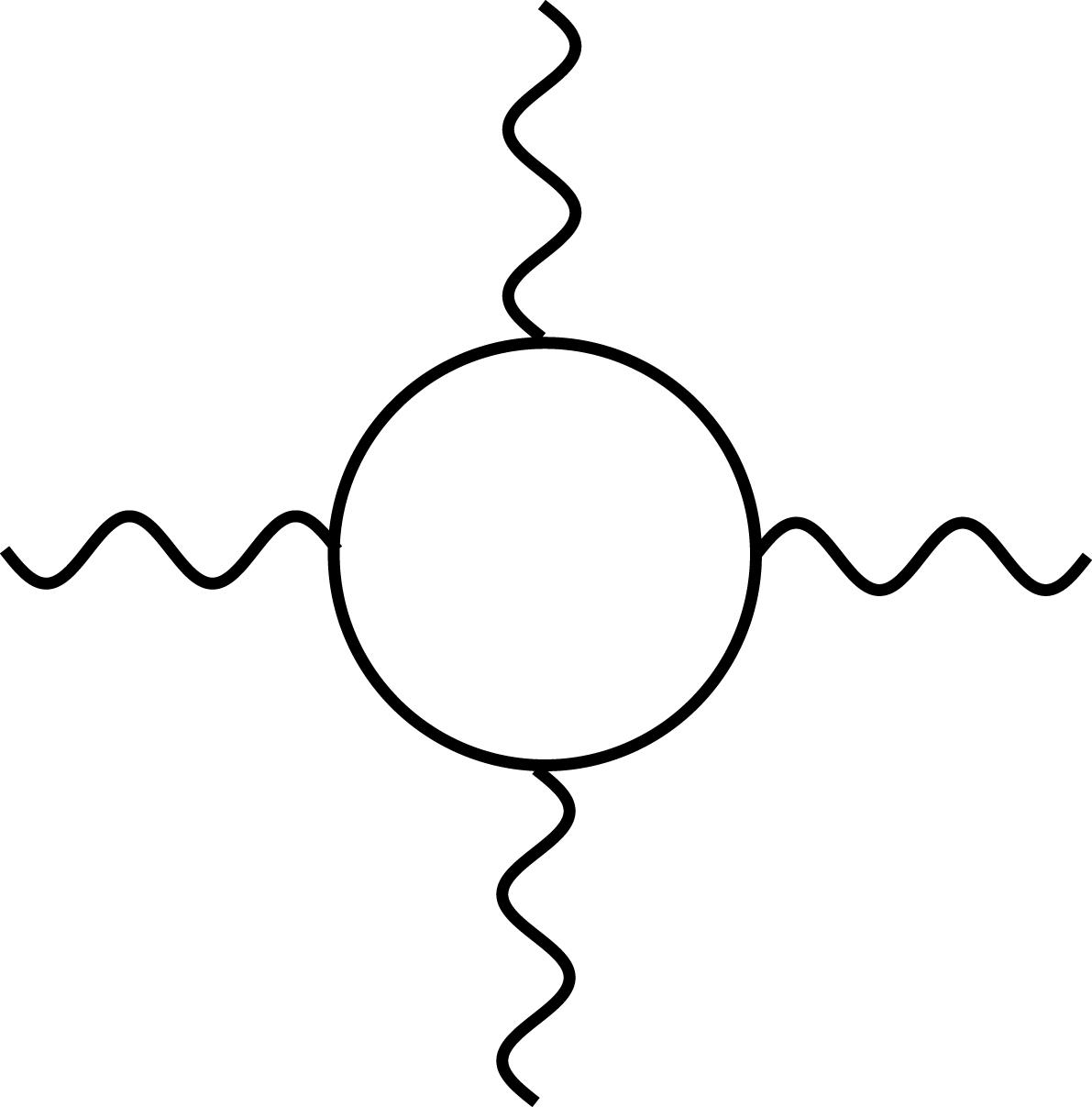
Fig. 2. Probing vacuum polarization by photon–photon scattering.
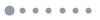
Fig. 3. The leading-order Heisenberg–Euler vertex or photon–photon scattering at low energies.
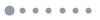
Fig. 4. A probe photon (wavy lines) scattering off a classical laser background (dashed lines) at low energy (so that the Heisenberg–Euler vertex can be employed).
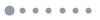
Fig. 5. Photons from the pump (dashes) interact with those from the probe to produce a pump-dependent vacuum index of refraction.
![Predicted diffracted electric field in a collision of two counterpropagating Gaussian beams. Adapted from [47].](/Images/icon/loading.gif)
Fig. 6. Predicted diffracted electric field in a collision of two counterpropagating Gaussian beams. Adapted from [47].
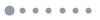
Fig. 7. Parametric frequency upshifting (left) and downshifting (right) can occur between pump and probe through the vacuum interaction.
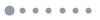
Fig. 8. Vacuum high-harmonic generation of the $n$ th harmonic of the probe via $2n$ -photon scattering.
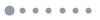
Fig. 9. Vacuum high-harmonic generation of the $n$ th harmonic of the probe via a chain of six-photon scattering.
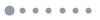
Fig. 10. An incoming probe photon can split into $k$ outgoing ones, due to interaction with the background.
![Cerenkov-like radiation (right) generated by pulse collapse into photon bullets (left) against longitudinal $z$ and transverse $r$ co-ordinates of an initially Gaussian pulse of central wavenumber $k_{0}$. Reproduced with permission[75].](/Images/icon/loading.gif)
Fig. 11. Cerenkov-like radiation (right) generated by pulse collapse into photon bullets (left) against longitudinal $z$ and transverse $r$ co-ordinates of an initially Gaussian pulse of central wavenumber $k_{0}$ . Reproduced with permission[75].
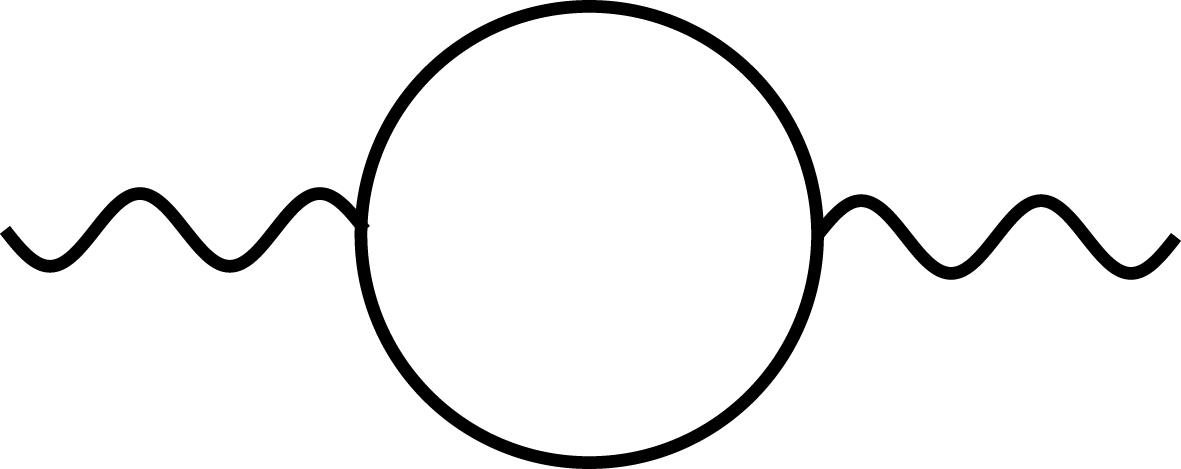
Set citation alerts for the article
Please enter your email address