Ruo-Jing Ren, Yong-Heng Lu, Ze-Kun Jiang, Jun Gao, Wen-Hao Zhou, Yao Wang, Zhi-Qiang Jiao, Xiao-Wei Wang, Alexander S. Solntsev, Xian-Min Jin, "Topologically protecting squeezed light on a photonic chip," Photonics Res. 10, 456 (2022)

Search by keywords or author
- Photonics Research
- Vol. 10, Issue 2, 456 (2022)
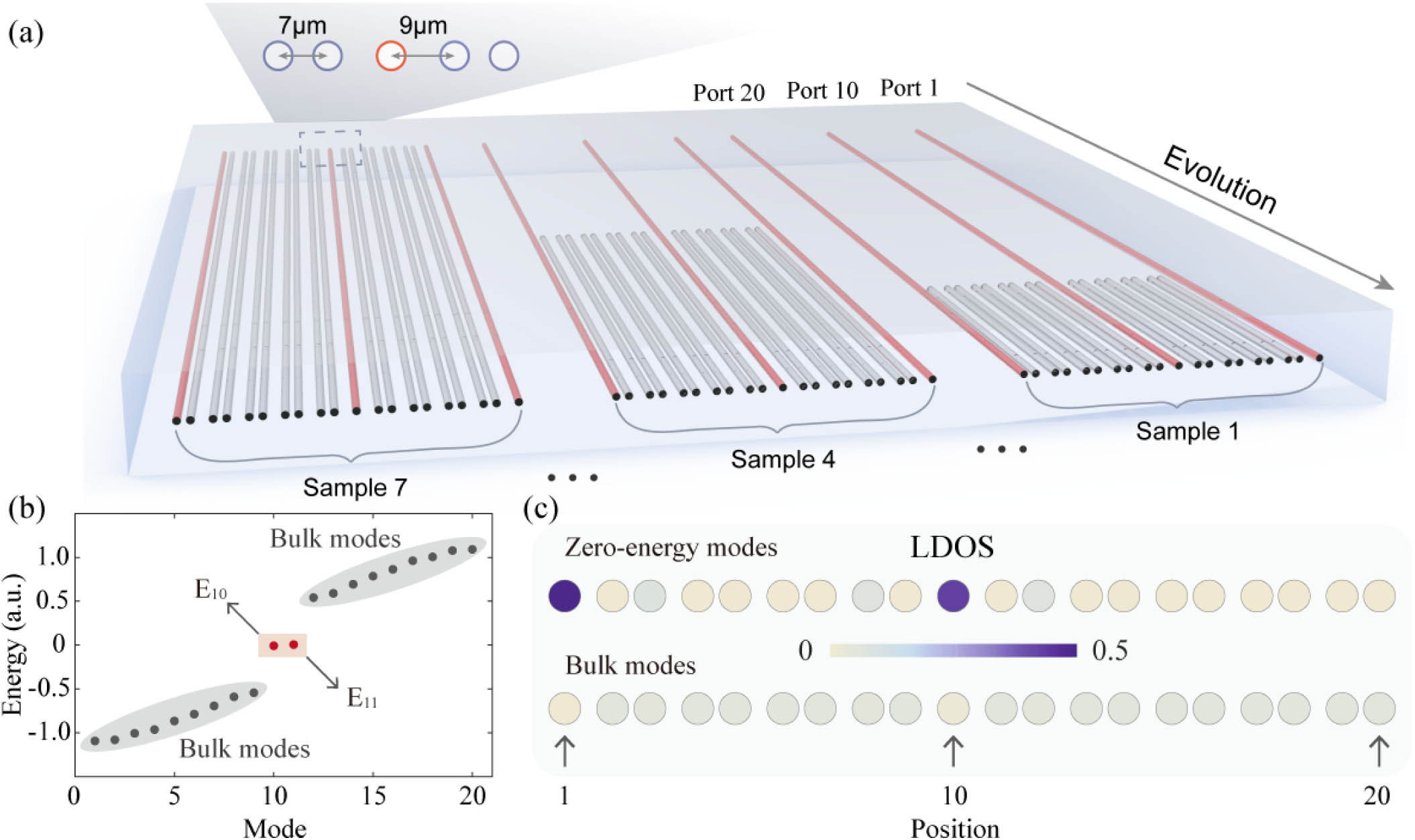
Fig. 1. Schematic diagram of the lattice with topological protection for squeezed light. (a) The structure of the lattice contains seven groups with lattice distances ranging from 5 mm to 35 mm. The short and long separations between adjacent waveguides are 7 μm and 9 μm, respectively. (b) Spectrum of the lattice. There are only two quasi zero-energy modes E 10 and E 11 and two extended bulk bands. Modes E 10 and E 11 are located inside the bandgap and decouple from the bulk bands, which promotes topological protection. a.u., arbitrary units. (c) Spatial distribution with combined local density of states (LDOS) for the quasi zero-energy modes E 10 and E 11 (upper line) marked by red in (b) and bulk modes (bottom line) marked by gray in (b).
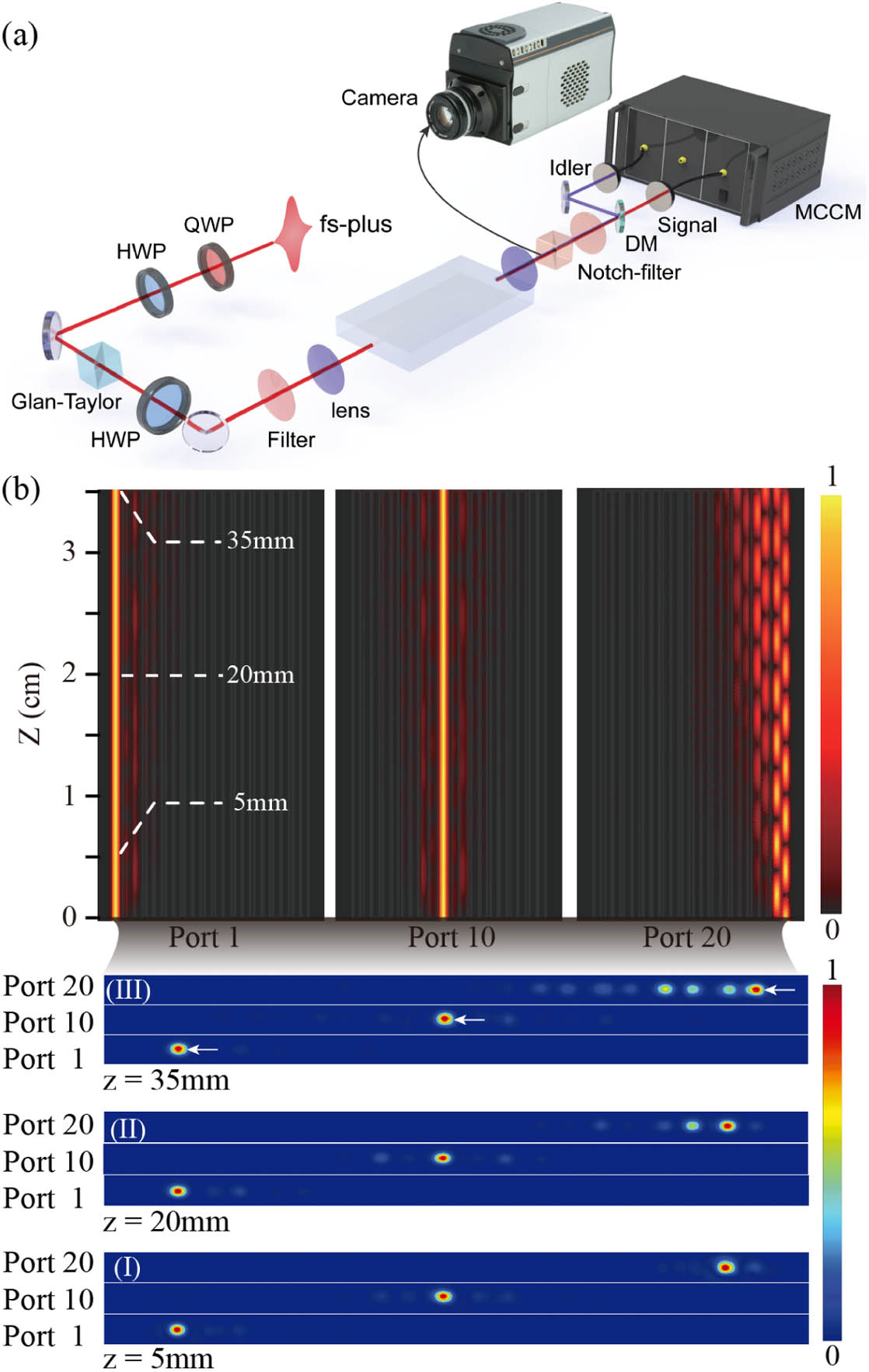
Fig. 2. Experimental setup and intensity distributions of pump light. (a) Experimental setup for generation, filtering, and evolution measurement of nonclassical light. The input ports can be switched. DM, dichroic mirror; MCCM, multi-channel coincidence module. (b) Simulated intensity distributions of the pump light from ports 1, 10, and 20. The evolution distance is marked in white. (I), (II), and (III) show the experimentally measured intensity distributions from three input channel ports at 5 mm, 20 mm, and 35 mm evolution distances, respectively. The protected states from ports 1 and 10 always maintain localization as the propagation distance increases, while the unprotected state from port 20 diffuses over the lattice. The intensity distribution is normalized to its maximum.
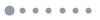
Fig. 3. Performance of topological protection for the generated photon pairs. (a) Evolution of the signal photons generated with pump in different input ports. (b) Evolution of the idler photons generated with pump in different input ports. (c) Cross-correlations g si 2 ( 0 ) with different input ports 1, 10, and 20 at different propagation distances from 5 mm to 35 mm with a step of 5 mm. The pump power is 1 mW with the integration time of 50 s. Cross-correlations g si 2 ( 0 ) of channel ports 1 and 10 are depicted in green and red, respectively, with high values, which demonstrates the topological protection of FWM. Cross-correlation g si 2 ( 0 ) in port 20 is depicted in blue, with the corresponding values being five times lower than the protected states from ports 1 and 10.
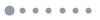
Fig. 4. Experimental verification of topological protection for squeezed light. The squeezing parameters in ports 1, 10, and 20 are depicted in deep red, red, and blue, accordingly. The values of the squeezing parameters are more uniform and higher in two protected cases compared with unprotected port 20. The variance among three topological channels is different since unprotected port 20 fluctuates in a wider range. The inset shows the average fluctuation from each port. Compared to the results of the other two channel ports, channel port 20 is unprotected.
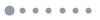
Fig. 5. Experimental schematic of measuring the heralded second-order correlation g H ( 2 ) ( 0 ) .
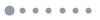
Fig. 6. Sketch of lattices for topological protection of squeezed light. There are 20 sites and 10 unit cells in the lattice. The red line marks the large spacing 9 μm with weak coupling of 0 . 3 mm − 1 , while the blue line marks the small spacing 7 μm with strong coupling of 0.8 mm − 1 .
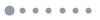
Fig. 7. Verification of topological protection effect provided by topological modules. We design two topological structures containing ports 1 and 10 from the main text, and a module containing port 20 is considered as a comparison. These modules are embedded into equally spaced arrays (d = 7 μm , d = 8 μm , and d = 9 μm ) to confirm the robustness and validity of topological protection. (a)–(c) Waveguide arrays inserted into different topological modules, namely, ports 1, 10, and 20. (d)–(f) Simulation result of the field distributions using three modules.
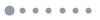
Fig. 8. Estimation of the mode field diameter. The intensity distributions of the light spots are extracted from the CCD data and fitted by 2D Gaussian functions. The beam diameters in x and y directions are calculated at the 1 / e 2 peak intensity points. We determine the scale of the figure by comparing the separation in pixel numbers and real waveguides. The final estimated results of horizontal and vertical field diameters are ( 5.466 ± 0.389 ) μm and ( 5.207 ± 0.217 ) μm , respectively.
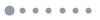
Fig. 9. Measured spectra of signal and idler photons.
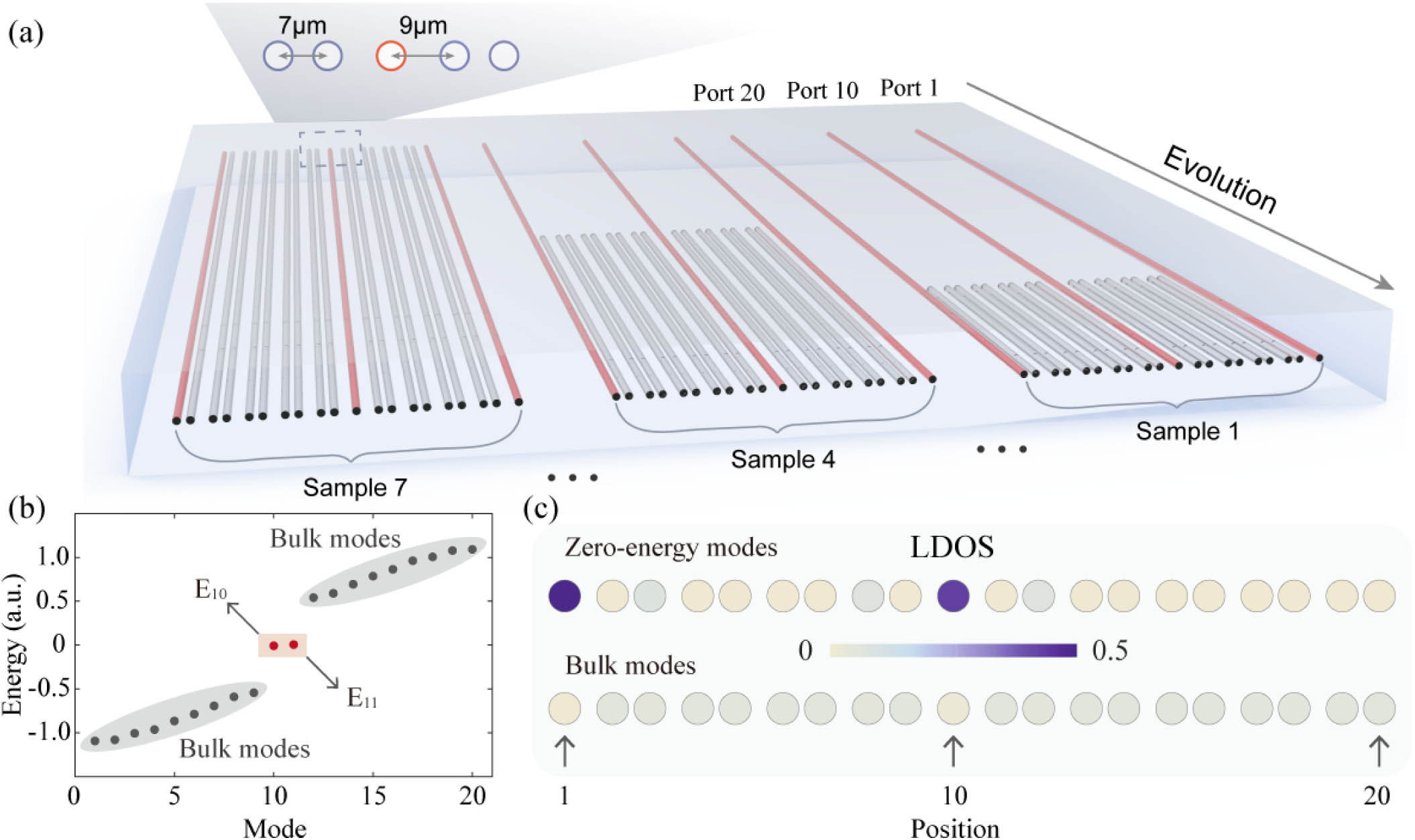
Set citation alerts for the article
Please enter your email address