Author Affiliations
1Hunan Key Laboratory for Super-Microstructure and Ultrafast Process, School of Physics and Electronics, Central South University, Changsha 410083, China2Printable Electronics Research Center, Suzhou Institute of Nano-Tech and Nano-Bionics, Chinese Academy of Sciences, Suzhou 215123, China3College of Engineering and Computer Science, Australian National University, Canberra 2600, Australia4Center for Excellence in Nanoscience, Key Laboratory of Nanosystem and Hierarchical Fabrication (CAS), National Center for Nanoscience and Technology, Beijing 100190, China5Changchun Institute of Applied Chemistry, Chinese Academy of Sciences, Changchun 130022, China6College of Chemistry and Chemical Engineering, Central South University, Changsha 410083, Chinashow less
In short, we found that the morphology and device performance for doctor-bladed PM6:Y6 thin films from low-boiling-point solvent are almost independent on the temperatures if controlling the ambient temperature and substrate temperature at the same value. With a designated illumination area of 26.75 cm2, the large-area PM6:Y6 modules demonstrate a certified PCE of 13.56%, and the PM6:Y6:PC61BM modules demonstrate a certified record PCE of 14.34%.
Organic solar cells (OSCs) have been developed rapidly in past years, due to the fast evolution of wide-bandgap copolymer donors and low-bandgap non-fullerene acceptors[1-9]. At present, the highest power conversion efficiencies (PCEs) for single-junction OSCs and tandem OSCs exceed 19% and 20%, respectively[10,11]. These OSCs are typically fabricated by using low-boiling-point solvent chloroform (CF) with an effective area <0.1 cm2. The doctor-blading deposition is the most advantageous technique to fabricate OSCs with low-boiling-point solvent for upscaling lab cells to industrial-scale modules[12], exhibiting simple operation, low cost, and high material utilization[13-15]. Herein, a typical OSC material system PM6:Y6 (Fig. 1(a)) was used to fabricate OSCs modulesvia doctor-blading deposition in ambient condition, and the influence of the ambient temperature and substrate temperature on the film quality was investigated. Furthermore, PC61BM was incorporated into PM6:Y6 blend, and a certified record efficiency of 14.34% was achieved for OSC modules with a designated illumination area of 26.75 cm2.
Furthermore, doctor-bladed large-area OSC modules were made at 24 °C in ambient condition. TheJ–V curves of the modules with a designated illumination area of 26.75 cm2 are shownFig. 1(e), and the photovoltaic parameters are listed inTable 1. The modules gave a PCE of 13.71%, with aVoc of 10.73 V, aJsc of 1.792 mA/cm2, and an FF of 71.29%. The certified PCE is 13.56% by the Chinese National PV Industry Measurement and Testing Center (NPVM) (Figs. S4–S10). Using fullerene derivatives is a simple and effective way to enhance PCE[22-24]. Thus, it is possible to further improve the module efficiencyvia introducing PC61BM into PM6:Y6 blend. The modules based on PM6:Y6:PC61BM (1 : 1 : 0.2) offer a record PCE of 14.35%, with aVoc of 10.89 V, aJsc of 1.814 mA/cm2, and an FF of 73.42% (Table 1). NPVM demonstrates a PCE of 14.34% (Figs. S11–S17). This certified PCE is the highest value reported to date for large-area OSC modules.
Some works indicated that the performance parameters for doctor-bladed OSCs from low-boiling-point solvent CF were normally dependent on the temperatures (25–45 °C)[12,16-18]. However, controlling the ambient temperature and substrate temperature at the same value, for example, from 17 to 30 °C, interesting results were achieved,i.e., doctor-bladed film quality and device performance are almost independent on temperature. Atomic force microscopy (AFM) images of doctor-bladed PM6:Y6 films at different temperatures (17, 24 and 30 °C) are almost the same (Fig. S1). Meanwhile, the root-mean-square roughnesses (Rrms) are around 1.50 nm. In addition, grazing incidence wide-angle X-ray scattering (GIWAXS) patterns for doctor-bladed PM6:Y6 thin films at different temperatures also exhibit the similar line-cut profiles (Fig. 1(b) and Fig. S2). The (010) peaks at 1.73 Å–1 in the out-of-plane (OOP) direction and the (100) peaks at 0.29 Å–1 in the in-plane (IP) direction indicate the co-existence of face-on and edge-on orientation in three cases. The diffraction peaks of PM6:Y6 films locate at the sameq values, indicating the same lamellar stacking distance and π–π stacking distance.
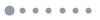
Figure 1.(Color online) (a) Chemical structures of PM6 and Y6. (b) 1D GIWAXS line curves along the out-of-plane (OOP, dotted line) and in-plane (IP, solid line) directions. (c)J–V curves for PM6:Y6 cells made at different temperatures. (d) EQE spectra for PM6:Y6 cells made at different temperatures. (e)J–V curves for PM6:Y6 and PM6:Y6:PC61BM modules with a designated illumination area of 26.75 cm2.
Doctor-bladed cells at three temperatures were fabricated with a structure of ITO/ZnO:PEI/PM6:Y6/MoO3/Ag[19-21]. The current density–voltage (J–V) curves and external quantum efficiency (EQE) spectra are shown inFigs. 1(c) and1(d), and the performance data are listed in Table S1. The cells processed at 17, 24 and 30 °C exhibit PCEs of 14.57%, 14.78% and 14.64%, respectively, and the average PCEs are 14.35%, 14.58% and 14.46%, respectively. The results suggest that the performance parameters for three cases are almost the same, which are consistent with the results of morphology and GIWAXS. The dependence ofJ–V characteristics on light intensity (Plight) was used to investigate the recombination kinetics in cells. As shown in Fig. S3(a), three cells show an almost equalα value of 0.975, indicating that the bimolecular recombination is not remarkable in PM6:Y6 cells made at three temperatures. TheVoc as a function ofPlight is plotted in Fig. S3(b). When the slope ofVoc versus ln(Plight) is equal to 1 or 2kT/q, the dominant recombination mechanism is bimolecular recombination or Shockley-Real-Hall recombination, respectively. The calculated slopes for the devices at 17, 24 and 30 °C are 1.398, 1.387 and 1.414kT/q, respectively. The results indicate that PM6:Y6 cells processed at three temperatures have relatively strong trap-assisted recombination, instead of bimolecular recombination.
Appendix A. Supplementary materials
Supplementary materials to this article can be found online athttps://doi.org/10.1088/1674-4926/43/10/100501.
Table Infomation Is Not Enable
References
[1] Y Lin, J Wang, Z G Zhang et al. An electron acceptor challenging fullerenes for efficient polymer solar cells. Adv Mater, 27, 1170(2015).
[2] J Yuan, Y Zhang, L Zhou et al. Single-junction organic solar cell with over 15% efficiency using fused-ring acceptor with electron-deficient core. Joule, 3, 1140(2019).
[3] Q Liu, Y Jiang, K Jin et al. 18% efficiency organic solar cells. Sci Bull, 65, 272(2020).
[4] J Qin, L Zhang, C Zuo et al. A chlorinated copolymer donor demonstrates a 18.13% power conversion efficiency. J Semicond, 42, 010501(2021).
[5] P Li, X Meng, K Jin et al. Banana-shaped electron acceptors with an electron-rich core fragment and 3D packing capability. Carbon Energy(2022).
[6] X Meng, M Li, K Jin et al. A 4-arm small molecule acceptor with high photovoltaic performance. Angew Chem Int Ed, e202207762(2022).
[7] J Cao, L Yi, L Ding. The origin and evolution of Y6 structure. J Semicond, 43, 030202(2022).
[8] J Cao, G Nie, L Zhang et al. Star polymer donors. J Semicond, 43, 070201(2022).
[9] Ding L. Organic solar cells. Wiley, 2022
[10] L Zhu, M Zhang, J Xu et al. Single-junction organic solar cells with over 19% efficiency enabled by a refined double-fibril network morphology. Nat Mater, 21, 656(2022).
[11] Z Zheng, J Wang, P Bi et al. Tandem organic solar cell with 20.2% efficiency. Joule, 6, 171(2022).
[12] A Distler, C J Brabec, H J Egelhaaf. Organic photovoltaic modules with new world record efficiencies. Prog Photovoltaics Res Appl, 29, 24(2021).
[13] W Guan, D Yuan, J Wu et al. Blade-coated organic solar cells from non-halogenated solvent offer 17% efficiency. J Semicond, 42, 030502(2021).
[14] M Li, J Wang, L Ding et al. Large-area organic solar cells. J Semicond, 43, 060201(2022).
[15] W Pan, Y Han, Z Wang et al. Over 1 cm2 flexible organic solar cells. J Semicond, 42, 050301(2021).
[16] R Sun, Q Wu, J Guo et al. A layer-by-layer architecture for printable organic solar cells overcoming the scaling lag of module efficiency. Joule, 4, 407(2020).
[17] B Zhang, F Yang, S Chen et al. Fluid mechanics inspired sequential blade-coating for high-performance large-area organic solar modules. Adv Funct Mater, 32, 2202011(2022).
[18] L Zhang, S Yang, B Ning et al. Modulation of vertical component distribution for large-area thick-film organic solar cells. Sol RRL, 6, 2100838(2022).
[19] X Guo, H Li, Y Han et al. Fully doctor-bladed efficient organic solar cells processed under ambient condition. Org Electron, 82, 105725(2020).
[20] H Li, K Huang, Y Dong et al. Efficient organic solar cells with the active layer fabricated from glovebox to ambient condition. Appl Phys Lett, 117, 133301(2020).
[21] Y Yang, E Feng, H Li et al. Layer-by-layer slot-die coated high-efficiency organic solar cells processed using twin boiling point solvents under ambient condition. Nano Res, 14, 4236(2021).
[22] K Jin, Z Xiao, L Ding. 18.69% PCE from organic solar cells. J Semicond, 42, 060502(2021).
[23] X Meng, K Jin, Z Xiao et al. Side chain engineering on D18 polymers yields 18.74% power conversion efficiency. J Semicond, 42, 100501(2021).
[24] G Zhang, X K Chen, J Xiao et al. Delocalization of exciton and electron wavefunction in non-fullerene acceptor molecules enables efficient organic solar cells. Nat Commun, 11, 3943(2020).