Aiwang Huang, Danni Chen, Heng Li, Dexiang Tang, Bin Yu, Jia Li, Junle Qu, "Three-dimensional tracking of multiple particles in large depth of field using dual-objective bifocal plane imaging," Chin. Opt. Lett. 18, 071701 (2020)

Search by keywords or author
- Chinese Optics Letters
- Vol. 18, Issue 7, 071701 (2020)
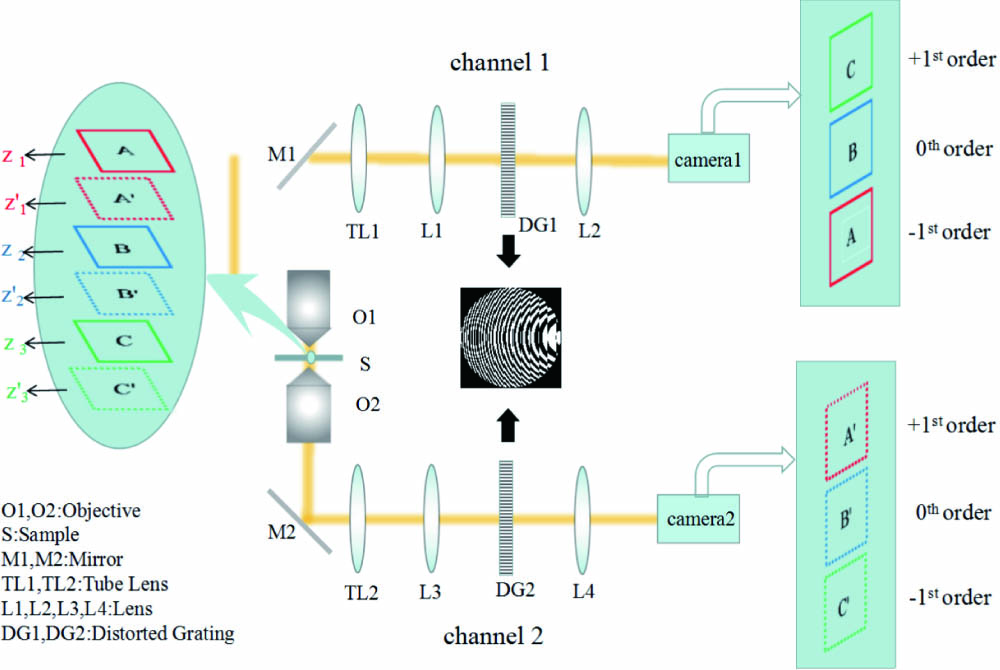
Fig. 1. Schematic diagram of DDBCM setup. The signal from particles in the sample (S) is collected by two identical objectives (O1, O2), resulting in two detection channels. In each channel, the signal passes through a tube lens (TL1 in channel 1 and TL2 in channel 2), then is modulated with a relay system consisting of two lenses (L1 and L2 in channel 1 and L3 and L4 in channel 2), where a distorted grating (DG1 in channel 1 and DG2 in channel 2) is mounted at the Fourier plane, and is finally detected by a camera (camera 1 in channel 1 and camera 2 in channel 2). As is shown in the enlarged sample area, three focal planes at depths , , and , denoted by capital letters A, B, and C for channel 1 (or at depths , , and , denoted by capital letters , , and for channel 2) separated by 4 μm are simultaneously imaged in three different areas, corresponding to three different diffraction orders. In channel 1, A, B, and C are simultaneously imaged on camera 1, while , , and in channel 2 are simultaneously imaged on camera 2.
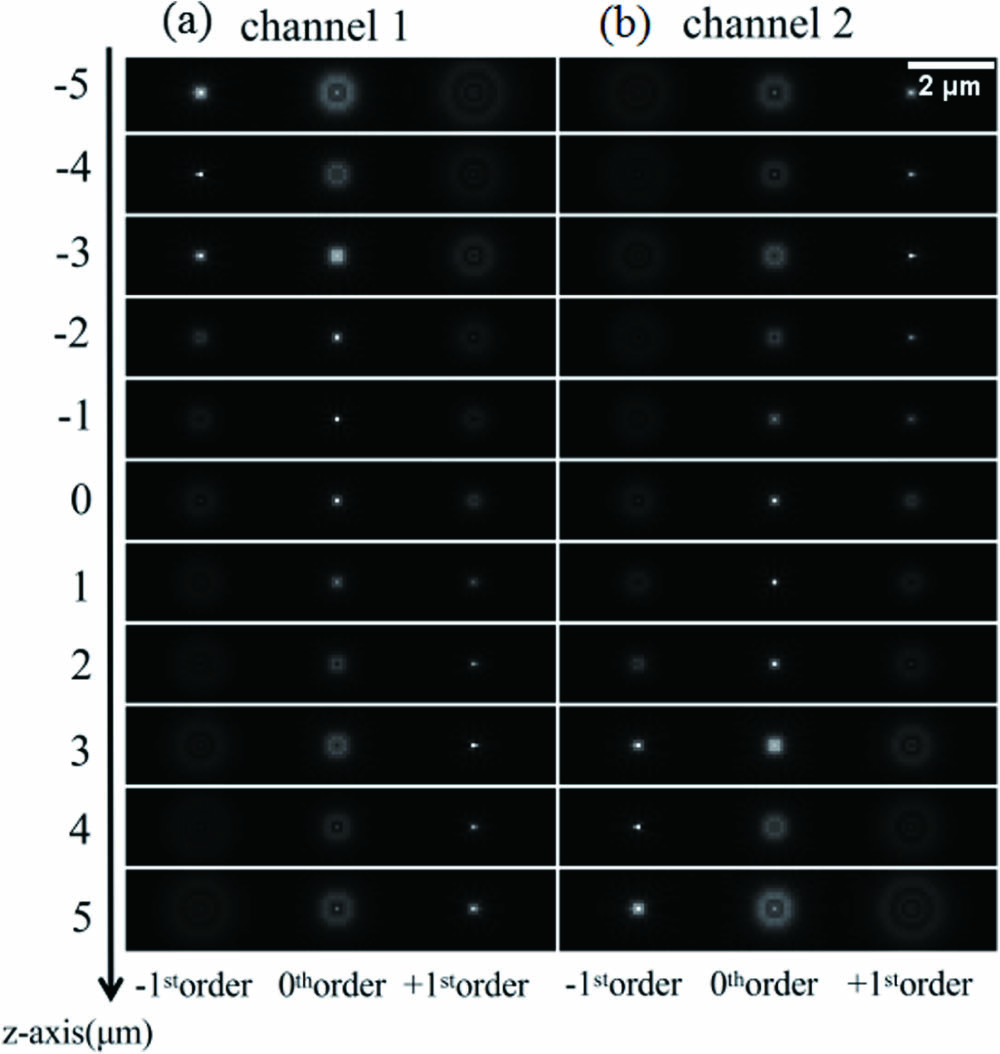
Fig. 2. Images of a single particle at 11 axial positions, from to . At each axial position, the particle is imaged in six areas, corresponding to three sub-imaging areas for the , , and diffraction orders in channel 1 and three other ones in channel 2. No matter where the particle is, it can be always captured in certain sub-images in the two channels.
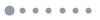
Fig. 3. Two trajectories of two particles. (a) Trajectories of P1 (bottom-up) and P2 (top-down) with time coding by the pseudo-color. (b) Three pairs of images at three time points, , 2.5 s, 5.0 s. For each particle, the image in each channel consists of three sub-images, which are from three different diffraction orders, respectively, and two appropriate sub-images are chosen for subsequent localization. The sub-images chosen for localization at the three time points are shown in blue and red circles.
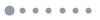
Fig. 4. Localization precision analysis of DDBCM. (a) 3D trajectories of the two particles, whose localizations were measured every 0.1 s from to , and the result localizations are shown with small circles. (b) Statistical localization precision along three directions. Gaussian fitting to the distribution of localization discrepancy of P1 at 101 time points demonstrated that the localization precisions in the , , and axes are 4.5 nm, 4.3 nm, and 17.1 nm, respectively. (c) Lateral ( and ) and axial ( ) localization precisions of the same particle throughout a depth range of 10 μm with the SNR set to 30 dB. (d) Localization precision in the , , and axes of the same particle at as a function of SNR.
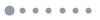
Fig. 5. Comparison of the (a) lateral and (b) axial localization precision and (c) the capability of 3D localization for the DDBCM approach, the biplane approach, and the DSBCM approach, whose detection strategies are shown in the left column. In all cases, each objective is assumed to collect 3000 photons for each particle, all these photons are evenly assigned to each sub-image, and the background level is set to 2 photons/pixel.
|
Table 1. Sub-images Chosen for 3D Localization Algorithm for Different Depth Ranges
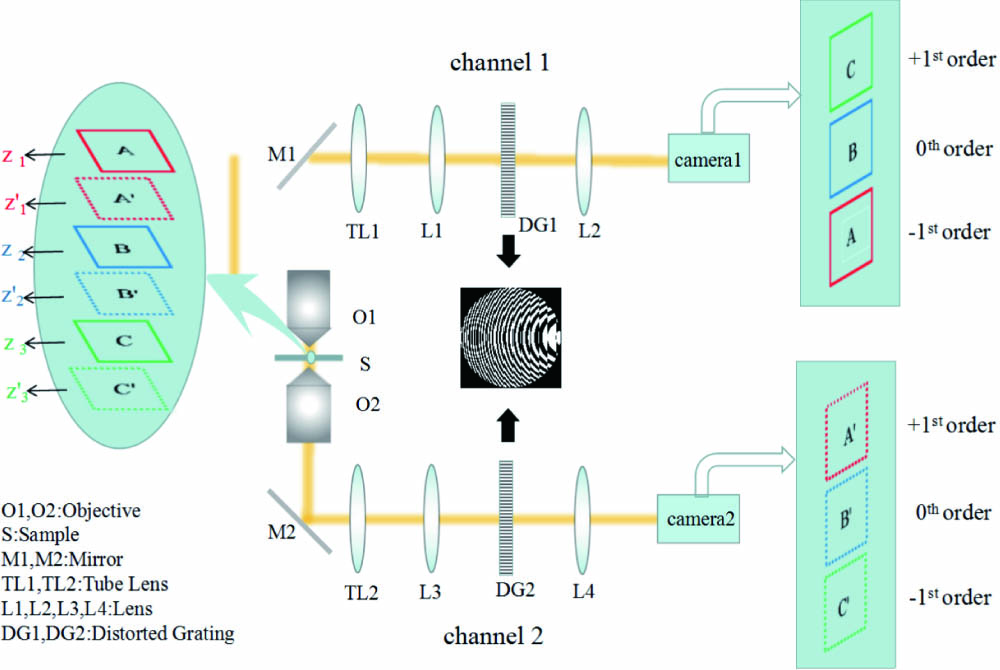
Set citation alerts for the article
Please enter your email address