
- Journal of Geographical Sciences
- Vol. 30, Issue 12, 2002 (2020)
Abstract
1 Introduction
The Tibetan Plateau (TP), with a mean elevation greater than 4000 m above sea level, plays a key role in modulating Asian and global climate systems (e.g.,
Although aeolian sand and loess deposits serve as an important archive for observing topographic and climatic events on the TP, the specific palaeoclimatic interpretations they reflect remain elusive. Generally, the formation of aeolian sediments requires three prerequisites: (1) availability of sediments for dust formation, (2) suitable wind for dust transportation, and (3) a suitable site for dust accumulation (
Local dust storms, sand dunes, and well-preserved aeolian sand and loess deposits are widespread in the southern TP (
On the other hand,
Previous studies have shown that the TP is predominantly controlled by the interplay between the ISM and the westerlies (
2 Material and methods
The YZR, with a length of 2,057 km, is the longest river in the southern TP; it lies between the Gangdise and Nyainqentanglha mountains and the Himalayas orogeny (
Figure 1.
In this study, we sampled five aeolian profiles, named DRX, RM, WL, SK, and DPZ (Figures 1 and 2), in the YZR valley and obtained four OSL ages (
Figure 2.
Sample | Depth (cm) | U (ppm) | Th (ppm) | K (%) | Dose rate (Gy•ka‒1) | De/Gy | OSL age (ka) |
---|---|---|---|---|---|---|---|
DRX-OSL | 68-72 | 1.56±0.3 | 13.95±0.7 | 2.41±0.04 | 3.73±0.27 | 2.93±0.30 | 0.79±0.10 |
RM-OSL-1 | 28-32 | 2.64±0.039 | 17.8±0.025 | 2.46±0.026 | 4.36±0.32 | 7.99±0.40 | 1.83±0.29 |
RM-OSL-3 | 208-212 | 3.09±0.038 | 19.2±0.024 | 2.06±0.030 | 4.17±0.31 | 19.35±0.98 | 4.64±0.36 |
DPZ-OSL-3 | 436-440 | 4.16±0.034 | 18.2±0.025 | 2.36±0.028 | 4.76±0.35 | 8.37±0.42 | 1.76±0.18 |
Table 1.
OSL dating results of aeolian sediments in the YZR basin
Sample | Depth/cm | Dating material | Conventional 14C age (BP, 2σ) | Calibrated14C age (BP, 2σ) |
---|---|---|---|---|
SK-14C-1 | 435-439 | Charcoal | 2880±25 | 3005±37 |
WL-14C-1 | 50-55 | Total organic matter | 710±20 | 670±6 |
Table 2.
Radiocarbon dating results of aeolian sediments in the YZR basin
Pure quartz was extracted in a dark room with a dimmed red light for OSL dating. Optically stimulated luminescence measurements were carried out on an automated Risø TL/OSL-DA-20-C/D reader, and De values were calculated with the single aliquot regenerative-dose (SAR) protocol (
To show the regional patterns of aeolian activity, we also considered prior published ages of aeolian profiles in the middle reaches of the YZR. Ultimately, we obtained a dataset that comprises ages of aeolian sand and loess in the middle reaches of the YZR, including 52 ages from 28 profiles (
Section | Depth (cm) | Dating method | Dating material | Age (ka/cal ka BP) | Latitude (°N) | Longitude (°E) | Altitude (m asl) | Source |
---|---|---|---|---|---|---|---|---|
TB1 | 350 | OSL | Aeolian loess | 2.70±0.20 | 29.3167 | 89.5500 | 3800 | |
TB7 | 380 | OSL | Aeolian loess | 11.00±1.20 | 29.3167 | 88.9167 | 3920 | |
DAR1 | 300-325 | 14C | Charcoal | 3.15±0.08 | — | — | — | |
STA1 | 50 | OSL | Aeolian sand | 2.90±0.20 | 29.6331 | 91.0978 | 3660 | |
STA1 | 180 | OSL | Aeolian sand | 4.10±0.40 | 29.6331 | 91.0978 | 3667 | |
STA1 | 280 | OSL | Aeolian sand | 6.70±0.50 | 29.6331 | 91.0978 | 3667 | |
QUX 1 | 280 | OSL | Aeolian sand | 8.50±0.70 | 29.3553 | 90.7234 | 3603 | |
QUX 2 | 325-330 | 14C | Charcoal | 7.78±0.07 | 29.3659 | 90.7556 | 3536 | |
Section 48 | 67-73 | OSL | Aeolian loess | 8.80±3.90 | 29.7333 | 89.8167 | 4571 | |
Section 49 | 47-53 | OSL | Aeolian loess | 7.80±1.20 | 29.7667 | 89.8500 | 4835 | |
LXD | 170 | OSL | Aeolian loess | 7.90±0.90 | 29.3275 | 89.5386 | 3797 | |
LXD | 98 | OSL | Aeolian loess | 3.20±0.30 | 29.3275 | 89.5386 | 3797 | |
Xigaze | — | 14C | Organic matter | 0.92±0.02 | 29.3057 | 88.8688 | 3811 | |
TDD | 87 | OSL | Aeolian loess | 2.60±0.30 | 29.3372 | 90.3236 | 3687 | |
TDD | 195 | OSL | Aeolian loess | 2.90±0.30 | 29.3372 | 90.3236 | 3687 | |
TDD | 285 | OSL | Aeolian loess | 5.00±0.50 | 29.3372 | 90.3236 | 3687 | |
JB | 260 | TL | Aeolian sand | 8.56±0.65 | 29.3969 | 89.3500 | 3890 | |
QS | 430 | TL | Aeolian loess | 8.85±0.53 | 29.3900 | 90.7578 | 4000 | |
GM | 340 | 14C | Organic matter | 6.20±0.31 | — | — | — | |
GM | 531 | TL | Aeolian sand | 8.30±0.30 | — | — | — | |
Cha'er | 65 | 14C | Organic mattera | 2.23±0.10 | 29.3895 | 89.2823 | 3856 | |
Cha'er | 235 | TL | Aeolian sand | 8.56±0.65 | 29.3895 | 89.2823 | 3856 | |
ZD | 158 | OSL | Aeolian loess | 5.90±0.20 | 29.2466 | 91.7120 | 3561 | |
ZD | 628 | OSL | Aeolian sand | 8.50±0.60 | 29.2466 | 91.7120 | 3561 | |
CGG | 168 | OSL | Aeolian sand | 1.82±0.16 | 29.3653 | 91.1491 | 3652 | |
CGG | 287 | OSL | Aeolian sand | 8.43±0.66 | 29.3653 | 91.1491 | 3652 | |
YJP1 | 0.4 | OSL | Sandy loess | 1.90±0.10 | 29.4556 | 94.4693 | 2943 | |
YJP1 | 0.9 | OSL | Sandy loess | 3.90±0.30 | 29.4556 | 94.4693 | 2943 | |
YJP1 | 1.4 | OSL | Sandy loess | 4.40±0.30 | 29.4556 | 94.4693 | 2943 | |
YJP1 | 1.9 | OSL | Sandy loess | 4.30±0.30 | 29.4556 | 94.4693 | 2943 | |
YJP1 | 2.5 | OSL | Sandy loess | 5.10±0.40 | 29.4556 | 94.4693 | 2943 | |
YJP1 | 3 | OSL | Sandy loess | 3.20±0.20 | 29.4556 | 94.4693 | 2943 | |
YJP1 | 3.6 | OSL | Sandy loess | 8.30±0.60 | 29.4556 | 94.4693 | 2943 | |
YJP2 | 1.7 | OSL | Sandy loess | 110±0.90 | 29.4556 | 94.4693 | 2943 | |
MLP | 6.5 | OSL | Aeolian sand | 4.50±0.30 | 29.1189 | 93.7781 | 3004 | |
MLP | 10 | OSL | Aeolian sand | 6.20±0.50 | 29.1189 | 93.7781 | 3004 | |
LXP | 1.3 | OSL | Sandy loess | 4.90±0.40 | 29.0668 | 92.7993 | 3172 | |
LXP | 2 | OSL | Sandy loess | 6.50±0.50 | 29.0668 | 92.7993 | 3172 | |
SRP | 0.7 | OSL | Aeolian sand | 0.40±0.10 | 29.2617 | 91.9873 | 3553 | |
Section | Depth (cm) | Dating method | Dating material | Age (ka/cal ka BP) | Latitude (°N) | Longitude (°E) | Altitude (m asl) | Source |
SRP | 1.4 | OSL | Aeolian sand | 0.80±0.10 | 29.2617 | 91.9873 | 3553 | |
SRP | 2.1 | OSL | Aeolian sand | 1.00±0.10 | 29.2617 | 91.9873 | 3553 | |
SRP | 2.8 | OSL | Aeolian sand | 1.10±0.10 | 29.2617 | 91.9873 | 3553 | |
SRP | 3.5 | OSL | Aeolian sand | 1.00±0.10 | 29.2617 | 91.9873 | 3553 | |
SRP | 4.2 | OSL | Aeolian sand | 1.20±0.10 | 29.2617 | 91.9873 | 3553 | |
SRP | 4.9 | OSL | Aeolian sand | 4.10±0.40 | 29.2617 | 91.9873 | 3553 | |
LCP | 2.9 | OSL | Sandy loess | 9.20±0.80 | 29.3872 | 89.3254 | 3815 | |
DRX | 70 | OSL | Aeolian sand | 0.79±0.10 | 29.3664 | 91.1494 | 3656 | This study |
RM | 30 | OSL | Aeolian loess | 1.83±0.29 | 29.3528 | 88.4615 | 3876 | This study |
RM | 210 | OSL | Aeolian loess | 4.64±0.36 | 29.3528 | 88.4615 | 3876 | This study |
WL | 52 | 14C | Organic matter | 0.67±0.01 | 29.1445 | 93.6766 | 3092 | This study |
SK | 437 | 14C | Charcoal | 3.01±0.04 | 29.2995 | 91.4115 | 3557 | This study |
DPZ | 438 | OSL | Aeolian sand | 1.76±0.18 | 29.2835 | 91.6485 | 3584 | This study |
Table 3.
A dataset of synthesized ages of Holocene aeolian sediments in the middle reaches of the YZR
3 Results and discussion
The cumulative probability density curve of the ages of Holocene aeolian sediments in the middle reaches of the YZR, as shown in
Figure 3.
Several events of increased dust deposition in the middle reaches of the YZR, such as the event around 8 ka, have not been reported on the northeastern TP (e.g.,
To gain a better understanding of the controlling factors of aeolian sedimentation in the middle reaches of the YZR, we compared our records with regional palaeoclimatic records. Reconstructed aeolian activities identified in this study are generally consistent with regional palaeohydrological records on the TP. Monsoon rainfall record collected from Paru Co, which is located in the southern TP, shows that several intervals of increased dust deposition mostly happen in the dry seasons (
Modern precipitation isotope observations have shown that moistures transported by the ISM can invade the TP through the Brahmaputra/YZR valley and reach as far as the Tanggula Mountains (
Different views exist on the main factors that control aeolian deposition on the TP. The formation of aeolian sediments requires/depends on the availability of dust sources, wind transportation, and suitable environment for dust deposition (
4 Conclusions
In this paper, we constructed a probability density curve of the ages of aeolian sand and loess in the middle reaches of the YZR, reflecting regional aeolian sediment accumulation intensity. We achieved this using a dataset that comprises new and prior published ages of Holocene aeolian sand and loess. In contrast to related studies on the northeastern TP, we found weak aeolian activities during the early Holocene in this region, and this may be associated with intensified ISM rainfall as a result of high summer insolation. Our results showed several intervals of increased dust accumulation centered at 8.5-7.8, 6.4-5.8, 4.5-4.0, 3.1-1.8, and 0.9 ka. These events are consistent with regional low rainfall events and weak ISM events. Regional monsoon rainfall/wetness, dominated by ISM activity, may play a key role in modulating dust emissions and aeolian deposition in the middle reaches of the YZR at centennial timescales. Overall, this study shows that aeolian deposits in the monsoon dominated TP can provide valuable information on past ISM activities. In the future, more effects of ISM activity would be identified with the availability of more data on the ages of aeolian sediments.
References
[2] AnZ, Kutzbach JE, Prell WL et al. Evolution of Asian monsoons and phased uplift of the Himalaya-Tibetan Plateau since Late Miocene times. Nature, 411, 62-66(2001).
[3] BergerA, Loutre MF. Insolation values for the climate of the last 10 million years. Quaternary Science Reviews, 10, 297-317(1991).
[4] Bird BW, Polisar PJ, LeiY. A Tibetan lake sediment record of Holocene Indian summer monsoon variability. Earth and Planetary Science Letters, 399, 92-102(2014).
[5] B?hnerJ. General climatic controls and topoclimatic variations in Central and High Asia. Boreas, 35, 279-295(2006).
[7] ChenF, WuD, ChenJ. Holocene moisture and East Asian summer monsoon evolution in the northeastern Tibetan Plateau recorded by Lake Qinghai and its environs: A review of conflicting proxies. Quaternary Science Reviews, 154, 111-129(2016).
[9] ChuG, SunQ, YangK et al. Evidence for decreasing South Asian summer monsoon in the past 160 years from varved sediment in Lake Xinluhai, Tibetan Plateau. Journal of Geophysical Research, 116, 1-11(2011).
[29] LiS, YangP, DongY et al. Desertification and Its Control in Tibet(2010).
[30] LiT, RenX, LiaoY. Paleoenvironment analysis of the middle reaches of Yarlung Zangbo River and Changguogou site. Quaternary Sciences, 40, 547-555(2020).
[32] LingZ, JinJ, WuD et al. Aeolian sediments and their paleoenvironmental implication in the Yarlung Zangbo catchment (southern Tibet, China) since MIS3. Acta Geographica Sinica, 74, 2385-2400(2019).
[33] LingZ, YangS, WangX et al. Spatial-temporal differentiation of eolian sediments in the Yarlung Tsangpo catchment, Tibetan Plateau, and response to global climate change since the Last Glaciation. Geomorphology, 357, 107104(2020).
[34] LiuX, DongH, YangX. Late Holocene forcing of the Asian winter and summer monsoon as evidenced by proxy records from the northern Qinghai-Tibetan Plateau. Earth and Planetary Science Letters, 280, 276-284(2009).
[35] LuH, ZhaoC, MasonJ. Holocene climatic changes revealed by aeolian deposits from the Qinghai Lake area (northeastern Qinghai-Tibetan Plateau) and possible forcing mechanisms. Holocene, 21, 297-304(2011).
[36] MaQ, ZhuL, LüX. Pollen-inferred Holocene vegetation and climate histories in Taro Co, southwestern Tibetan Plateau. Chinese Science Bulletin, 59, 4101-4114(2014).
[37] MaQ, ZhuL, LüX. Late glacial and Holocene vegetation and climate variations at Lake Tangra Yumco, central Tibetan Plateau. Global and Planetary Change, 174, 16-25(2019).
[39] MolnarP, Boos WR, Battisti DS. Orographic controls on climate and paleoclimate of Asia: Thermal and mechanical roles for the Tibetan Plateau. Annual Review of Earth and Planetary Sciences, 38, 77-102(2010).
[40] Murray AS, Wintle AG. Application of the single-aliquot regenerative-dose protocol to the 375°C quartz TL signal. Radiation Measurements, 32, 579-583(2000).
[41] PanM, WuY, ZhengY. Holocene aeolian activity in the Dinggye area (southern Tibet, China). Aeolian Research, 12, 19-27(2014).
[42] Prescott JR, Hutton JT. Cosmic ray contribution to dose rates for luminescence and ESR dating: Large depths and long-term time variations. Radiation Measurements, 23, 497-500(1994).
[43] PyeK. The nature, origin and accumulation of loess. Quaternary Science Reviews, 14, 653-667(1995).
[44] QiangM, JinY, LiuX. Late Pleistocene and Holocene aeolian sedimentation in Gonghe Basin, northeastern Qinghai-Tibetan Plateau: Variability, processes, and climatic implications. Quaternary Science Reviews, 132, 57-73(2016).
[45] QiangM, LiuY, JinY. Holocene record of eolian activity from Genggahai Lake, northeastern Qinghai-Tibetan Plateau, China. Geophysical Research Letters, 41, 589-595(2014).
[46] Reimer PJ, BardE, BaylissA. IntCal13 and Marine13 radiocarbon age calibration curves 0-50,000 years cal BP. Radiocarbon, 55, 1869-1887(2013).
[47] SekiO, Meyers PA, YamamotoS. Plant-wax hydrogen isotopic evidence for postglacial variations in delivery of precipitation in the monsoon domain of China. Geology, 39, 875-878(2011).
[48] ShenW, LiH, SunM. Dynamics of aeolian sandy land in the Yarlung Zangbo River basin of Tibet, China from 1975 to 2008. Global and Planetary Change, 86/87, 37-44(2012).
[49] ShiX, KirbyE, Furlong KP. Rapid and punctuated Late Holocene recession of Siling Co, central Tibet. Quaternary Science Reviews, 172, 15-31(2017).
[50] SinghviA, BluszczA, BatemanM. Luminescence dating of loess-palaeosol sequences and coversands: Methodological aspects and palaeoclimatic implications. Earth-Science Reviews, 54, 193-211(2001).
[51] StauchG. Geomorphological and palaeoclimate dynamics recorded by the formation of aeolian archives on the Tibetan Plateau. Earth-Science Reviews, 150, 393-408(2015).
[52] StauchG. Multi-decadal periods of enhanced aeolian activity on the north-eastern Tibet Plateau during the last 2 ka. Quaternary Science Reviews, 149, 91-101(2016).
[53] StauchG, IjmkerJ, P?tschS. Aeolian sediments on the north-eastern Tibetan Plateau. Quaternary Science Reviews, 57, 71-84(2012).
[54] StauchG, LaiZ, LehmkuhlF. Environmental changes during the late Pleistocene and the Holocene in the Gonghe Basin, north-eastern Tibetan Plateau. Palaeogeography, Palaeoclimatology, Palaeoecology, 509, 144-155(2018).
[55] SunJ, LiS, Muhs DR. Loess sedimentation in Tibet: Provenance, processes, and link with Quaternary glaciations. Quaternary Science Reviews, 26, 2265-2280(2007).
[57] TianL, YaoT, MacCluneK. Stable isotopic variations in west China: A consideration of moisture sources. Journal of Geophysical Research: Atmospheres, 112, 1-12(2007).
[58] Tian LD, Masson-DelmotteV, StievenardM. Tibetan Plateau summer monsoon northward extent revealed by measurements of water stable isotopes. Journal of Geophysical Research Atmospheres, 106, 28081-28088(2001).
[63] ZhangJ, Feng JL, HuG. Holocene proglacial loess in the Ranwu valley, southeastern Tibet, and its paleoclimatic implications. Quaternary International, 372, 9-22(2015).
[64] Zhang JF, XuB, TurnerF et al. Long-term glacier melt fluctuations over the past 2500 yr in monsoonal High Asia revealed by radiocarbon-dated lacustrine pollen concentrates. Geology, 45, 359-362(2017).
[67] ZhengY. The response of Holocene aeolian activities to climate change in the typical area of Qinghai-Tibet Plateau: Take the broad valley area in the middle reaches of the Yarlung Zangbo River in south Tibet and the Gonghe basin in Qinghai as examples. Beijing: Beijing Capital Normal University(2009).
[68] ZhengY, WuY, LiS. Grain-size characteristics of sediments formed since 8600 yr BP in middle reaches of Yarlung Zangbo River in Tibet and their paleoenvironmental significance. Chinese Geographical Science, 19, 113-119(2009).
[69] ZhuL, LiY, LiB. The ostracod assemblages and their environmental significance in the Chen Co area, southern Tibet in recent 1400 years. Journal of Geographical Sciences, 12, 451-459(2002).
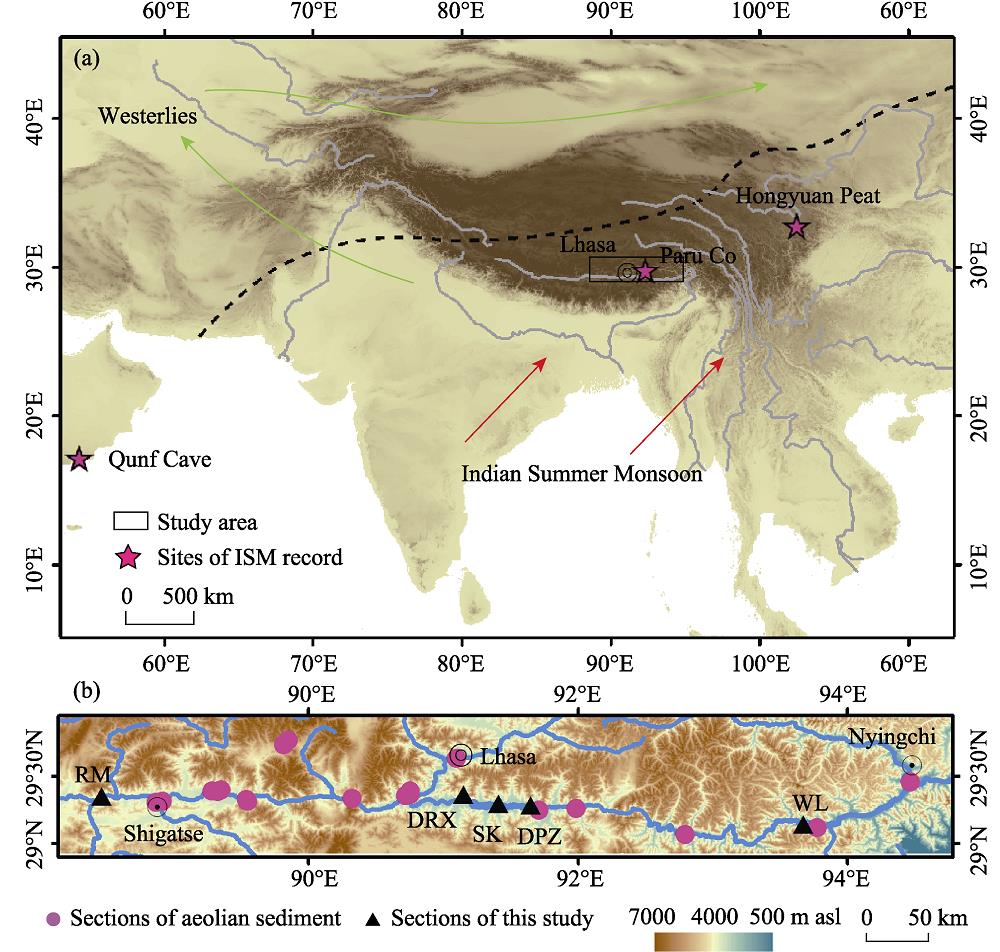
Set citation alerts for the article
Please enter your email address