Author Affiliations
1CAS Key Laboratory of Quantum Information, University of Science and Technology of China, Hefei 230026, China2CAS Center For Excellence in Quantum Information and Quantum Physics, University of Science and Technology of China, Hefei 3006, China3Department of Physics, Ocean University of China, Qingdao 266100, Chinashow less
Fig. 1. The scheme of extremal semiquantum nonlocal game. Players (Alice and Bob) share state ρ^AB, and then, they receive quantum questions τ^iA0, ω^iB0 from referee (Charlie). After performimg local POVM on their own part of shared state and the quantum question state, they return classical answers x and y to Charlie. LOCC is allowed in this game. If the shared state is entangled, then Alice and Bob can always win this game (achieve payoff value larger than 0) as long as they perform suitable measurements.
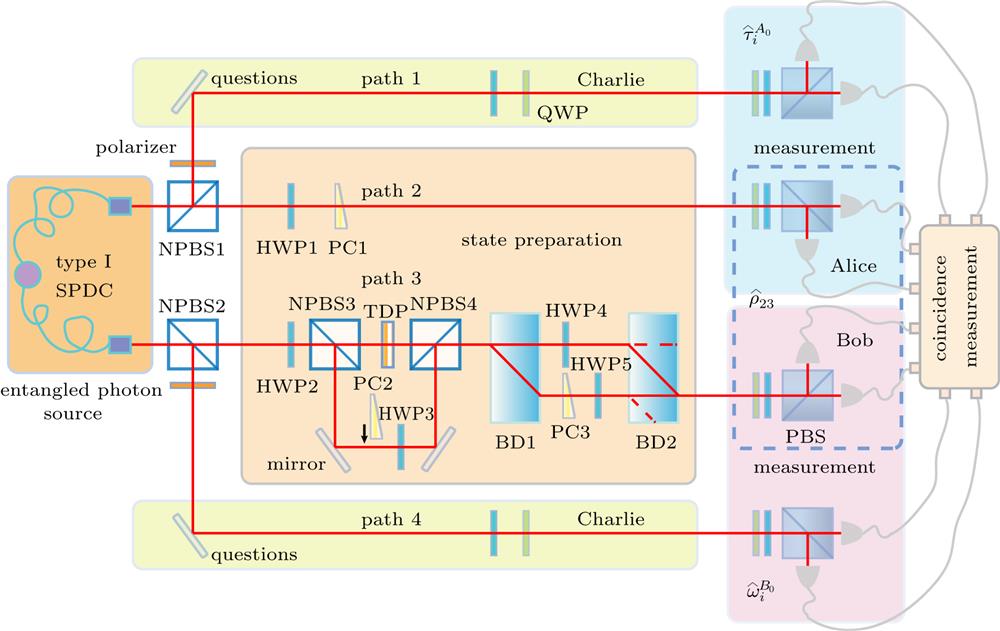
Fig. 2. Experimental setup for MDI-UEW. The original entangled photon pairs are generated by type-I SPDC in two 2-mm BBO crystals with the form of 12(|HV〉+eiϕ|VV〉). The reflected parts of non-polarizing beam splitters NPBS1 and NPBS2 are used as question states. The transmitted parts of NPBS1 and NPBS2 are used to prepare ρ^AB. By selecting a suitable phase compensator PC1, |Φ+〉 or |Φ−〉 can be prepared. When half wave-plate HWP2 is set at an angle of 45°, and HWP1 is set at an angle of 0°, |Ψ+〉, |Ψ−〉 can be prepared. The bypath of NPBS3 is used to prepare the second Bell state component in the Bell diagonal state. HWP4 and HWP5 are used to change the ratio between H-polarization and V-polarization in |Φ+〉, then a redundant amount of H- or V-polarization will be discarded when passing through beam displacer BD2. Then states cos θ|HH〉 + sin θ|VV 〉 are prepared. Wave-plate combinations in path 1 and path 4 are used to rotate the polarizations to encode photons 1 and 4 to the desired question states, τ^iA0 and ω^iB0. The measurement part consists of four QWP-HWP-PBS combinations, where QWP and PBS denoting quarter wave-plate and polarizing beam splitter, respectively. All the four photons are separately detected by single-photon avalanche diodes, but the four-photon events are coincidently counted together and analyzed by a picosecond time analyzer with well-tuned delays.
![Comparison between the MDI-UEW and CEW on entanglement detection ability and comparison between the EWM and concurrence on entanglement quantification ability. CEW is calculated by Tr(W^0ρ^). EWM represents E(ρ^), which is the witness outcome of MDI-UEW. (a) Experimental results for the Bell states. The related collections of Bell states are labelled in the parentheses. The orange bars represent the experimental results of the EWM, the green bars represent the CEW, and the blue bars represent the estimated concurrence. (b) Experimental results of cos θ|HH〉 + sin θ|VV〉 (θ ∈ [0,π/2]). The theoretical values of the EWM (EWMth, red dashed line) and concurrence (Con.th, black solid line) are both sin 2θ, while the theoretical value of CEW (blue solid line) is –1. Red circular points represent the experimental results of EWM (EWMexp), whereas the black square points represent the estimated concurrence (Con.exp). Blue triangle points represent the results of CEW without any ability for entanglement detection or quantification for the target states.](/Images/icon/loading.gif)
Fig. 3. Comparison between the MDI-UEW and CEW on entanglement detection ability and comparison between the EWM and concurrence on entanglement quantification ability. CEW is calculated by Tr(W^0ρ^). EWM represents E(ρ^), which is the witness outcome of MDI-UEW. (a) Experimental results for the Bell states. The related collections of Bell states are labelled in the parentheses. The orange bars represent the experimental results of the EWM, the green bars represent the CEW, and the blue bars represent the estimated concurrence. (b) Experimental results of cos θ|HH〉 + sin θ|VV〉 (θ ∈ [0,π/2]). The theoretical values of the EWM (EWMth, red dashed line) and concurrence (Con.th, black solid line) are both sin 2θ, while the theoretical value of CEW (blue solid line) is –1. Red circular points represent the experimental results of EWM (EWMexp), whereas the black square points represent the estimated concurrence (Con.exp). Blue triangle points represent the results of CEW without any ability for entanglement detection or quantification for the target states.
Fig. 4. Concurrence and EWM for a series of asymmetric states. States are defined in Eq. (7), the two parameters (p,θ) are united as x, which is p = 0.01x and θ = 0.01xπ. EWM of a state is less than or equal to its concurrence.
States | p | EWMth. | EWMexp. | CEWth. | CEWexp. |
---|
State 1 | 0.7 | 0.4 | 0.393 ± 0.029 | 0.4 | 0.393 ± 0.033 | State 2 | 0.55 | 0.1 | 0.076 ± 0.031 | –1 | –0.980 ± 0.020 | State 3 | 0.4 | 0.2 | 0.173 ± 0.031 | –0.2 | –0.211 ± 0.027 |
|
Table 1. Experimental results for the Bell diagonal states.