Abstract
A metal-lined hollow-core fiber-based Raman probe extension kit is proposed in this Letter for in situ and sensitive ultramicro-analysis. A hollow-core fiber can confine light and fluid samples in its hollow core, with enhanced light–sample interaction. By using a homemade light coupling device with a glass window for liquid isolation, a 3.5-cm-long hollow-core fiber could mount on and connect to a Raman probe, with perfect light coupling efficiency. After full filling the hollow-core fiber chamber with a volume of 13 μL by using a syringe pump, it can act as an extension kit for an ordinary Raman probe and be used as a ultramicro-analysis tool for the sample of microfluidic chips. In order to enhance its sensitivity, a gold film coated fiber tip is inserted into the capillary, which can double the Raman signal received by reflecting pump light and Raman light. Finally, a detection limit of 5% for ethanol solution and an enhancement factor of two compared with direct detection of bulk sample volume are demonstrated. Above all, our device can be utilized as a Raman probe extension kit, which is suitable for rapid, sensitive, and in situ measurements for a few microliter level samples.Raman spectrometry based on molecular vibration and rotation provides information about the molecular structure of the sample to be detected. It could be treated as the unique fingerprint of the analyte and widely used to identify chemical components and molecules[1–3] in different domains including biosensor[4,5], food safety[6], and so on. Moreover, Raman technology is also important for on-site rapid inspection of chemicals, drugs, explosives, and food quality by using a portable Raman spectrometer and its probe[7]. However, a traditional portable Raman spectrometer always has very low sensitivity and can only detect pure and bulk samples with a long integration time[8]. Furthermore, in many special applications, such as for the case of very expensive or rare samples, the output of the micro total analysis system (), the sample volume that we can get is only a few microliters () or less, which makes it difficult for direct detection using a probe. So, there is a great demand for a portable Raman probe with high sensitivity for ultramicro-analysis.
For sensitivity improvement, several methods were proposed to solve the problem of detection limitation by using the localized field enhancement effect, such as surface-enhanced Raman scattering (SERS)[9] and tip-enhanced Raman scattering (TERS)[10]. However, due to the surface effect, a very small volume of sample attached on the hot spots may dominate the Raman signal harvest. So, there is serious cross contamination in the samples exchange, which limits its application in online detection. On the other hand, the hollow-core fiber, including hollow-core photonic crystal fibers (HC-PCF)[11,12] and metal-lined hollow-glass fibers/capillaries (MLHCFs)[13–15], can not only be a chamber of light–sample interaction, but also has excellent signal collection efficiency. Even through the HC-PCF has relatively high detection sensitivity due to its extremely low loss[16], it is not suitable for online detection since it requires high pressure pumping equipment for sample loading. On the other hand, MLHCFs give a better solution with advantages, including (1) larger hollow-core diameter of a few hundreds of micrometers; (2) faster sample exchange within a few seconds, even under normal pressure; (3) volumetric enhancement mechanism with less cross contamination; (4) being suitable for ultramicro-analysis with a few sample volume. However, its sensitivity needs to be improved due to relatively poor optical transmission efficiency compared with HC-PCF.
In this Letter, an MLHCF-based Raman probe extension kit is proposed, which could be used in ultramicro-analysis with improved sensitivity by inserting a gold film coated fiber tip into the MLHCF and reflecting pump and Raman light. Compared with direct detection using a bare probe, its sensitivity is improved by a factor of two. Further, the linear relationship between the concentration and intensity of the Raman signal and no cross contamination are demonstrated in the sample exchange. The total sample volume is as small as with an injection time of 5 s. Above all, with our device, a rapid, portable, real-time, and highly sensitive Raman detector can be achieved, which makes it possible to realize online detection.
Sign up for Chinese Optics Letters TOC. Get the latest issue of Chinese Optics Letters delivered right to you!Sign up now
A schematic sketch of our proposed probe extension kit is depicted in Fig. 1. Firstly, separated with a glass window for liquid isolation, the silver-lined capillary and the Raman probe are connected and mounted in sleeve A. The pump light emerging from the Raman probe couples into the inner core of the silver-lined capillary and reacts with the substance to stimulate Raman light. The silver-lined capillary confines the light and sample in its hollow core to enhance the light–sample interaction. On the other end of the silver-lined capillary, a glass rod coated with gold film is inserted into the capillary, and the structure is sealed in sleeve B. The gold film can enhance the received Raman signal by reflecting all of the pump laser and Raman light. Specifically, the gold film reflects the pump light to double its transmission distance in the silver-lined capillary, which enhances the Raman signal. Besides, it also reflects backward and forward scattering Raman light, which avoids Raman signal leaking away from the silver-lined capillary. In order to achieve better coupling efficiency, we have mounted eight screws on the four sides of sleeve A to slightly adjust the lateral position and tilt angle of the Raman probe. At last, the Raman signal is collected by the Raman probe. In a word, the Raman probe extension kit with perfect coupling efficiency and low transmission loss is highly sensitive for sample detection.
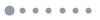
Figure 1.Schematic diagram of a portable Raman probe extension kit.
We have also realized a fluidic control system aimed at repeatability of the Raman detector. As is depicted in Fig. 1, both ends of the silver-lined capillary are connected to the sample and a syringe pump with Teflon tubes, respectively. As a result, we can control the sample in and out by pulling and pushing the syringe pump. Since the inner core of the capillary is larger than the HC-PCF, we realized the fast and easy injection of the sample that is required for in situ detection. Lastly, we packaged up the MLHCF-based Raman probe extension kit so that it is portable and can be applied into in situ detection.
In experiments, we assembled the MLHCF-based Raman probe extension kit as is shown in Fig. 2. Firstly, the Raman probe and the silver-lined capillary were mounted and immobilized in sleeve A using UV adhesive glue. The silver-lined capillary, which is 3.5 cm long, was purchased from Do-Ko Corporation with an inner diameter of and outer diameter of . The part of the silver-lined capillary inserted into the sleeve is protected by a ceramic ferrule. A glass cover slip was inserted in front of the Raman probe to prevent contamination of the sample. On the left of the Raman detector, the silver-lined capillary and ordinary capillary of similar size were inserted with the glass rod coated with gold film, as is depicted schematically in Fig. 1. The thickness of the gold film on the end of the glass rod is 200 nm, and the reflectance is 95%. While the ordinary capillary on the left was fixed with the glass rod by gluing, the silver-lined capillary on the right, where the sample can flow into it, was relatively movable to the glass rod. All the capillaries were inserted and immobilized in sleeve B by UV adhesive glue to hold the glass rod coated with gold film. Lastly, the left end of the ordinary capillary was sealed to prevent the sample from leaking out.
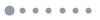
Figure 2.Experimental diagram of the portable Raman probe extension kit.
Besides, Teflon tubes connected sleeve A with a syringe pump and sleeve B with a microfluidic chip containing the sample, respectively. We operated the syringe pump to achieve the injection, cleaning, and replacement of the sample. When we pull the syringe, the sample will be absorbed into the silver-lined capillary. Then, we drain the sample in the capillary by pushing the syringe. In addition, we rinse the capillary with deionized water before and after injection of the sample to remove impurities. The liquid quantity required in the silver-lined capillary as the Raman reaction chamber at any time is as low as , and the injection time is about 5 s, which is necessary for ultramicro-analysis and fast detection.
The Raman signal received is analyzed by a Raman spectrometer (BWTEK i-Raman plus). The Raman spectra have been processed with adaptive iteratively reweighted penalized least squares (airPLS) algorithm for correcting the baseline, which eliminates the background[17].
In experiments, to demonstrate the improvement of the device detection capability, we have firstly tested the Raman spectrum of ethanol using a Raman probe directly to compare with the Raman detector silver-lined hollow-core fiber (SLHCF), as illustrated in Fig. 3. The power of the pump laser is 90 mW. The integration time is 2 s. The blue and orange lines represent the Raman spectra detected by our Raman detector and Raman probe, respectively. It is calculated that the intensity of the Raman signal received by the Raman detector is about two times of that received by direct detection. Therefore, the detection results demonstrated that the Raman-probe-based extension kit increased the sensitivity of the Raman probe.
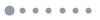
Figure 3.Comparison of the Raman spectra for ethanol detected by SLHCF and direct detection.
By reflecting pump light and Raman light, gold film inserted into the silver-lined capillary is important for the sensitivity of the detector. In experiments, we also explored the enhancement rate of the gold film by detecting the Raman signal utilizing the Raman detector. As is shown in Fig. 4, the blue and orange lines represent the Raman spectrum of anhydrous ethanol detected by the Raman detector with gold film and without gold film, respectively. The gold film enhanced the Raman signal by two times and improved the sensitivity of the Raman detector.
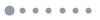
Figure 4.Comparison of the Raman spectra for ethanol detected by the Raman detection system with and without gold film.
Further, we detected the Raman spectra of ethanol solution, whose concentrations ranged from 5% to 100%, without altering the light coupling condition. Between successive detections, we rinsed the silver-lined capillary with deionized water to remove impurities. Detected by the Raman detector proposed, the Raman spectra of the ethanol solution in different concentrations with respect to water are demonstrated in Fig. 5(a). The detection limit of the Raman detector is 5% for the ethanol solution. We separated and demonstrated Raman spectra around (C-C-O band) with varied concentrations in the inset of Fig. 5(a). Further, the Raman peak area, which is integrated in the intensity of the Raman characteristic peak around , varied with the concentration of ethanol solution, as is depicted in the scatter diagram Fig. 5(b). Using the linear fitting method, the linear relationship between the Raman peak area and the concentration of ethanol solution can be obtained to be ( is the concentration of ethanol solution, S is the Raman peak area integrated around ). The linear fitting equation predicted the concentration accurately with of 0.9917. We have also obtained similar linear fitting results for other characteristic peaks, which further prove the linearity of the intensity of the Raman signal and the concentration of the sample.
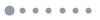
Figure 5.(a) Raman spectra for the ethanol solution whose concentration ranged from 5% to 100% with respect to water. (b) The linear fitting relationship between the Raman intensity at and the concentration for the ethanol solution.
Based on volumetric enhancement, the Raman detector proposed can avoid cross disruption, which the Raman technique based on SERS cannot overcome. SERS detection is so sensitive that it can detect very small amounts of liquid and amplify the Raman signal by several magnitudes. However, amplification may also introduce unintended noise from impurities. Even though the concentration of the impurities may be very low, the stimulated Raman signal may interrupt the Raman spectrum of the sample to be detected. However, based on volumetric enhancement, the Raman detector proposed excludes the disturbance from impurities. In experiments, to prove no cross disruption of the Raman detector proposed, we have tested the Raman spectra for ethanol solution and isopropanol solution in different concentrations with respect to water continuously. The solution of different concentrations was injected, detected, and cleaned continuously, as is depicted in Fig. 6(c). Between consecutive detections, we rinsed the capillary with deionized water to remove impurities, as is depicted in Fig. 6(d). The Raman spectra for ethanol and isopropanol are represented in Fig. 6(e), while the intensities at (C-C-O band) and (C-C-O band) are chosen to denote the composition and concentration of solution without mutual interference. Figures 6(a) and 6(b) represent the Raman intensity at for the ethanol solution and the Raman intensity at for the isopropanol solution detected at specific times. At around 200 s, the ethanol solution was replaced by isopropanol solution. No Raman detected signal at concludes that successive detections do not have cross disruption in the Raman detector proposed.
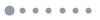
Figure 6.(a) The Raman intensity at for the ethanol solution in water with the concentration varied in time. (b) The Raman intensity at for the isopropanol solution in water with the concentration varied in time. (c) The concentration of the ethanol solution and isopropanol solution injected into the capillary varied with the time. (d) The schematic on the replacement and cleaning of the sample in experiments. (e) The Raman intensities at and are chosen to represent ethanol and isopropanol without mutual interference.
In this work, the Raman-enhanced detector based on hollow-core fiber is very suitable for online detection with the merits of sensitivity and portability. Besides, the Raman detector requires a very small amount of liquid, which means that it can be applied to many circumstances. For example, the Raman detector can be integrated with microfluidic chips to analyze the chemical composition in practical applications. Microfluidic chips where micro-liquid is controlled and reacts to generate samples in a tiny volume raise very high requirements for the Raman detector. Direct detection using the Raman probe, which requires large volumes of the sample, is unable to detect the sample in microfluidic chips. The Raman detector we proposed can satisfy the detection requirements. However, the proposed Raman probe extension kit can only be used to detect liquid samples, meaning that it cannot be generalized to the detection of gases.
In this Letter, an MLHCF-based Raman probe extension kit was proposed. In the homemade light coupling device, the Raman probe and the hollow-core fiber are mounted and have perfect coupling efficiency. Besides, the hollow-core fiber confining the light has low transmission loss. The gold film enhanced the Raman light by reflecting the pump light and Raman light twofold, which is proved in experiments. In addition, the Raman signal detected by the Raman detector increased by about two times when compared with direct detection. Furthermore, the detection limit of the device is 5% for ethanol solution. The linearity of the concentration of ethanol solution and the intensity of the Raman signal was proved to be . Above all, the Raman detector without cross disruption is highly sensitive for the sample to be detected. It can also realize the injection, cleaning, and replacement of the sample, while the volume of the sample in the silver-lined capillary is , and the injection time needed is 5 s. Therefore, the Raman detector can be integrated with microfluidic chips to apply to online detection and ultramicro-analysis, which cannot be realized by a Raman probe directly. Overall, the Raman-enhanced detection device can be utilized as a Raman probe extension kit that improves the performance of the Raman probe for rapid, sensitive, and in situ measurements.
References
[1] Y. Lu, X. Kan, T. Xu, M. Wang, C. Yin, J. Fang, X. Chen. Chin. Opt. Lett., 16, 012301(2018).
[2] A. Kudelski. Talanta, 76, 1(2008).
[3] C. Shi, X. Wang, P. Zhou, X. Xu. Chin. Opt. Lett., 15, 110605(2017).
[4] J. Fang, J. Fang, Z. Li. Biomaterials, 32, 4877(2011).
[5] K. Egodage, C. Matthäus, S. Dochow, I. W. Schie, C. Härdtner, I. Hilgendorf, J. Popp. Chin. Opt. Lett., 15, 090008(2017).
[6] A. P. Craig, A. S. Franca, J. Irudayaraj. Annu. Rev. Food Sci. Technol., 4, 369(2013).
[7] A. Nguyen, Z. D. Schultz. Analyst, 141, 3630(2016).
[8] S. D. Schwab, R. L. McCreery. Appl. Spectrosc., 41, 126.
[9] K. Kneipp, Y. Wang, H. Kneipp, L. T. Perelman, I. Itzkan, R. R. Dasari, M. S. Feld. Phys. Rev. Lett., 78, 1667(1997).
[10] W. Zhang, X. Cui, B. Yeo, T. Schmid, C. Hafner, R. Zenobi. Nano Lett., 7, 1401(2007).
[11] A. Khetani, J. Riordon, V. Tiwari, A. Momenpour, M. Godin, H. Anis. Opt. Express, 21, 12340(2013).
[12] S. Hanf, T. Bögözi, R. Keiner, T. Frosch, J. Popp. Anal. Chem., 87, 982(2015).
[13] Z. Jin, Q. Chu, W. Xu, H. Cai, W. Ji, G. Wang, B. Lin, X. Zhang. IEEE Photon. Technol. Lett., 30, 387(2018).
[14] T. M. James, S. Rupp, H. H. Telle. Anal. Methods, 7, 2568(2015).
[15] S. Rupp, A. Off, H. Seitz-Moskaliuk, T. M. James, H. H. Telle. Sensors, 15, 23110(2015).
[16] A. Khetani, A. Momenpour, E. I. Alarcon, H. Anis. Opt. Express, 6, 4599(2015).
[17] Z. Zhang, S. Chen, Y. Liang. Analyst, 135, 1138(2010).