Jonathan Peltier, Weiwei Zhang, Leopold Virot, Christian Lafforgue, Lucas Deniel, Delphine Marris-Morini, Guy Aubin, Farah Amar, Denh Tran, Xingzhao Yan, Callum G. Littlejohns, Carlos Alonso-Ramos, Ke Li, David J. Thomson, Graham Reed, Laurent Vivien, "High-speed silicon photonic electro-optic Kerr modulation," Photonics Res. 12, 51 (2024)

Search by keywords or author
- Photonics Research
- Vol. 12, Issue 1, 51 (2024)
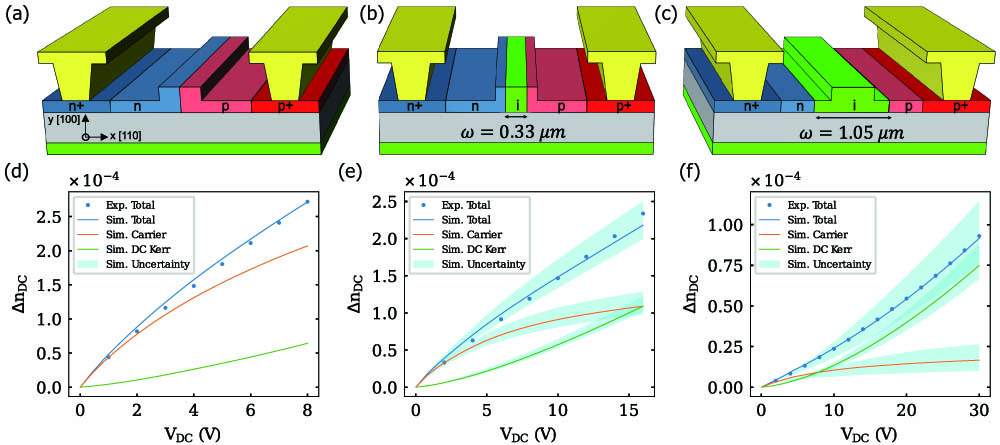
Fig. 1. (a) Depiction of PN junction, (b) PIN with intrinsic region width w = 0.33 μm (PIN2), and (c) PIN with w = 1.05 μm (PIN3). (d) Effective refractive index changes of PN, (e) PIN2, and (f) PIN3 junctions versus the applied reverse DC bias voltage with respective MZM arm lengths of 2, 6, and 6 mm. Dots are the experimental measurements, and lines correspond to the respective simulations of the whole modulation of the DC Kerr effect and carrier modulations.

Fig. 2. (a) Schematic view of the experimental setup used to measure the EOM from the MZM. DC voltage is applied to both arms; RF is either applied in single-drive or push–pull configuration (EDFA, erbium-doped fiber amplifier). (b) Effective index variations measured in push–pull configuration versus the reverse DC bias for a fixed RF peak amplitude of 1.4 V, (c) versus the RF amplitude for three reverse DC biases.
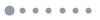
Fig. 3. Dots and the lines represent, respectively, the measurements and the corresponding fit or simulations. (a) Optical MZM transfer function for three electrical spectral components excluding intrinsic losses with P 0 the maximum output power, P DC the static power, P Ω the modulation power at angular frequency Ω , and P 2 Ω at frequency 2 Ω for the PIN3 junction by applying reverse V DC = 6 V , V RF = 2.0 V . (b) Amplitude of the refractive index modulation at angular frequency 2 Ω versus the applied voltage V RF at frequency Ω for reverse DC biases from 0 to 15 V. Whatever the value V DC , it induces no variation of Δ n 2 Ω . (c) Respective relative contribution of index variation in the Ω component from EFI linear EOM and from carrier modulation versus the applied reverse DC bias voltage.
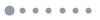
Fig. 4. (a) Setup used to acquire eye diagrams (PPG, pulse pattern generator; DC, reverse DC bias; EDFA, erbium-doped fiber amplifier; DCA, digital communications analyzer). (b) Extinction ratio and signal-to-noise ratio at 10 Gbit/s by applying dual 4V p p DATA / DATA ‾ driving in push–pull versus the applied reverse DC bias. Eye diagrams for reverse DC bias of 2 and 30 V are embedded.
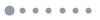
Fig. 5. Optical eye diagram display from 1 mm long PIN3 modulator by applying dual 2V p p DATA / DATA ‾ driving in push–pull and reverse V DC = 30 V measured at (a) 80 Gbit/s and (b) 100 Gbit/s with a numerical six taps feed-forward equalization (FFE).
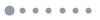
Fig. 6. Optical transmission of an unbalanced MZI for an applied reverse bias of 0 and 30 V. λ r is the resonance wavelength, FSR ( λ r ) the free spectral range, and Δ λ r the wavelength shift for bias voltage variation from 0 to 30 V.
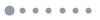
Fig. 7. Measurements (dots) of P DC , the MZM output DC optical power; P Ω , the modulation at angular frequency Ω ; P 2 Ω , the modulation at angular frequency 2 Ω as a function of wavelength for (a) the 2 mm long PN junction using V DC = 2 V , V RF = 0.51 V , (b) the 5 mm long PIN2 junction using V DC = 4 V , V RF = 1.6 V , and (c) the 5 mm long PIN3 junction using V DC = 6 V , V RF = 2.0 V . The dashed lines represent the corresponding fit.
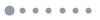
Fig. 8. Eye diagram, respectively, measured at 2, 10, 18, 30 V reverse DC bias at 10 Gbits/s using 4V p p on each arm corresponding to ER 1.5, 2.4, 3.3, and 3.7 dB, and SNR 8.9, 12.8, 14.3, 15.6 dB.
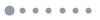
Fig. 9. Optical eye diagram of 6 mm long PIN3 modulator measured at a data rate of (a) 32 Gbit/s and (b) 40 Gbit/s using 4V p p on each arm and reverse V DC = 30 V , with ER 2.7 dB and 2.3 dB, respectively.
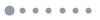
Fig. 10. Cross section of the fabricated device viewed with an SEM.
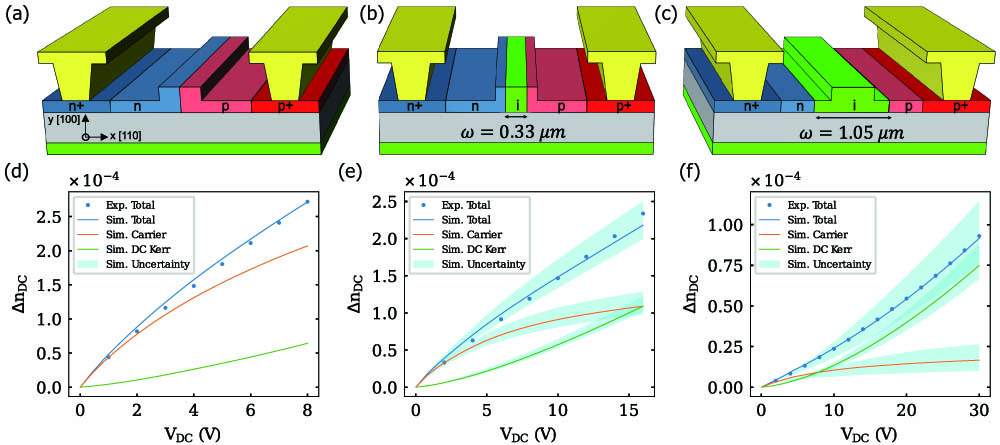
Set citation alerts for the article
Please enter your email address